
Therapeutic Potential of Silymarin in Inhibiting the Fibroblast-to-Myofibroblast Transition in Renal Interstitial Fibroblasts
Ⓒ The Society of Pathology in Korean Medicine, The Physiological Society of Korean Medicine
Abstract
Renal fibrosis (RF) is a prominent pathological feature of chronic kidney disease (CKD), characterized by excessive accumulation of extracellular matrix components, resulting in progressive renal function loss. The fibroblast-to-myofibroblast transition (FMT) plays a pivotal role in renal fibrosis pathogenesis, driving aberrant deposition of extracellular matrix proteins and disruption of tissue architecture. Targeting FMT has emerged as a promising strategy to combat renal fibrosis and preserve kidney function. Silymarin, a flavonoid extract derived from Silybum marianum seeds, has gained attention for its therapeutic potential, particularly in liver diseases, due to its potent antioxidant and anti-inflammatory properties. However, the precise mechanisms underlying its effects on FMT remain unclear. This study aimed to investigate the therapeutic potential of silymarin in inhibiting FMT in NRK-49F renal interstitial fibroblasts. Transforming growth factor-beta 1(TGF-β1) plays a crucial role in promoting FMT through the activation of intracellular signaling pathways and induction of key fibrotic markers, including alpha-smooth muscle actin (α-SMA) and vimentin. Silymarin demonstrated significant downregulation of FMT markers, including α-SMA and vimentin, in TGF-β1-stimulated NRK-49F cells. Our findings highlight silymarin as a promising therapeutic candidate for mitigating renal fibrosis and managing CKD.
Keywords:
TGF-β1, Tranforming growth factor beta 1, FMT, Fibroblast-to-myofibroblast transitionIntroduction
Chronic kidney disease (CKD) is a global health concern, affecting over 10-14 % of the population worldwide, with an estimated 800 million individuals affected. CKD is associated with significant morbidity and mortality and is characterized by the progressive loss of renal function1,2). Renal fibrosis (RF) is a common pathological feature observed in CKD, regardless of the initial injury or underlying disease3). It represents the final common pathway of CKD progression, leading to the disruption of renal architecture, loss of functional nephrons, and reduction in renal blood flow4). The prevalence of RF in CKD is substantial, contributing to the relentless decline in renal function. The excessive accumulation of extracellular matrix (ECM) components, including collagen, fibronectin, and proteoglycans, within the renal interstitium results in the loss of normal tissue architecture and impaired organ function. This fibrotic remodeling process hampers the regeneration and repair capacity of the kidney, ultimately leading to end-stage renal disease (ESRD) and the need for renal replacement therapy, such as dialysis or transplantation.
Despite the clinical significance of RF, effective therapeutic strategies to halt or reverse its progression remain limited. The development of effective therapies for RF is a complex task due to the multifactorial nature of the disease. Additionally, the heterogeneity of CKD etiologies adds further challenges to the development of targeted treatments5).
Fibroblast activation and their transition into myofibroblasts (FMT) play a crucial role in driving the excessive production of ECM proteins and tissue remodeling in RF6). In response to profibrotic stimuli, such as cytokines, and growth factors, quiescent fibroblasts undergo a phenotypic switch characterized by increased expression of contractile proteins, such as α-SMA, and enhanced synthesis and secretion of ECM components, including collagen, fibronectin, and proteoglycans7). The profibrotic cytokine, transforming growth factor-β (TGF-β), is a pivotal mediator of FMT, promoting the expression of α-SMA and vimentin, enhancing ECM synthesis, and inhibiting matrix degradation8). TGF-β triggers a cascade of intracellular events involving Smad-dependent and Smad-independent pathways, including mitogen-activated protein kinase (MAPK) and mammalian target of rapamycin (mTOR) signaling pathways9).
Silymarin, a natural compound extracted from the milk thistle plant (Silybum marianum), has been used for centuries as an herbal remedy for liver diseases due to its antioxidant, anti-inflammatory, and anti-fibrotic properties10,11). Silymarin has been also reported to possess chemoprotective properties, providing protection against ischemia-reperfusion injury and UV-induced immunosuppression12-14). Recent evidence suggests that silymarin may exert its anti-fibrotic effects on the kidney by inhibiting TGF-β signaling15). However, the effect of silymarin on the RF and its underlying mechanisms, particularly concerning FMT, has not been thoroughly investigated.
This study aims to elucidate the effect of silymarin on FMT, focusing on the expression of α-SMA and vimentin, and the related TGF-β signaling pathways. Our findings may shed light on the potential of silymarin as a therapeutic agent in the management of RF.
Materials and Methods
1. Cell Culture
NRK-49F cells (obtained from ATCC) were cultured in Dulbecco's Modified Eagle's Medium (DMEM) supplemented with 10% fetal bovine serum (FBS) and 1% penicillin-streptomycin (P/S) at 37℃ with 5% CO2. The medium was replaced every 2-3 days, and cells were sub-cultured at 80% confluency using trypsin-EDTA.
2. Chemicals, Reagents, and Antibodies
Silymarin, (purity > 98%) obtained from SIGMA-ALDRICH (St.Louis, MO, USA) was dissolved in dimethyl sulfoxide (DMSO) for experimental use. Primary antibodies against Vimentin and α-Smooth Muscle Actin (α-SMA) were obtained from Abcam (Cambridge, UK). Antibodies targeting JNK, phospho-JNK (Thr183), p38, phospho-p38 (Thr180), Smad2/3, phospho-Smad2/3 (Ser465/Ser467), p70S6K, and phospho-p70S6K (Thr389) were sourced from Cell Signaling Technology, Inc. (Beverly, MA, USA). Antibodies for GAPDH and horseradish peroxidase (HRP)-conjugated secondary antibodies were procured from Santa Cruz Biotechnology (Santa Cruz, CA, USA). Polyvinylidene difluoride (PVDF) membranes (Immobilon-P) were sourced from Millipore Co. (Billerica, MA, USA). The SuperSignal® West Pico chemiluminescent substrate was obtained from Pierce Biotechnology, Inc. (Rockford, IL, USA). Recombinant human Transforming Growth Factor-β1 (TGF-β1) was purchased from PEPROTECH Inc. (Rocky Hill, NJ, USA). The EZ-Cytox assay was purchased from DoGenBio (Seoul, Korea). The RNeasy Kit was purchased from Qiagen (Hilden, Germany), and the iScriptTM cDNA Synthesis Kit was obtained from Bio-Rad Laboratories, Inc. (Hercules, CA, United States). SYBR Green Real-Time PCR Master Mix was purchased from Thermo Fisher Scientific Inc. (Waltham, MA, USA). Unless otherwise specified, all additional chemicals and solvents were of analytical grade and procured from E. Merck or Sigma-Aldrich Co. The ProLong™ Gold Antifade Mountant with DAPI and Alexa Fluor 488 for immunofluorescence staining was purchased from Invitrogen (Carlsbad, CA, USA).
3. Cell viability
The cytotoxicity of silymarin was assessed through a meticulous protocol using the EZ-Cytox assay. The procedure began with the preparation of the cell culture, following which 100 μl of the culture was dispensed into each well of a 96-well plate. Upon completion of the pre-incubation phase (2 hours), 10 μl of silymarin, prepared at diverse concentrations, was added to each well for 72 hours. In the ensuing step, 10 μl of EZ-Cytox reagent was introduced into each well. Subsequent to the addition of EZ-Cytox, the plate was returned to the incubator for 30 min of reaction period. Finally, the absorbance was measured at 450nm using a plate reader, with a reference wavelength set between 600 and 650nm.
4. Western blotting
NRK-49F cells were lysed with RIPA buffer containing protease inhibitors, and the protein concentration of the lysates was determined using a BCA protein assay kit. Equal amounts of protein (10mg) were separated on a 9% SDS-PAGE gel and transferred to a PVDF membrane. The membrane was blocked with 5% non-fat milk in TBS-T buffer and incubated overnight at 4℃ with primary antibodies respectively. After washing with TBS-T buffer, the membrane was incubated with HRP-conjugated secondary antibody for 1 hour at room temperature. The protein bands were visualized using an ECL kit, and the band intensities were quantified using ImageJ software. Protein expression levels were normalized to those of GAPDH, which served as a loading control. All experiments were performed in triplicate.
5. Real-time PCR
To evaluate the impact of Silymarin on the mRNA expression levels of vimentin and α-SMA in TGF-β1 stimulated NRK-49F cells, we employed a real-time polymerase chain reaction (RT-PCR) approach. NRK-49F cells were pre-incubated with silymarin for 16 hours and subsequently exposed to TGF-β1 for 4 hours. RNA was then isolated from the cells using the RNeasy Kit, following the manufacturer's instructions. The isolated RNA was used as a template for complementary DNA (cDNA) synthesis, employing the iScriptTM cDNA Synthesis Kit, in accordance with the manufacturer's protocol. Subsequently, RT-PCR was performed for vimentin and α-SMA using Real-Time PCR Master Mix, following the provided instructions.
The primer sequences used in PCR were as follows: α-SMA forward, 5'-CATCACCAACTGGGACGACA-3' and reverse, 5'-TCCGTTAGCAAGGTCGGATG-3'; vimentin forward, 5'-CATGCGGCTGCGAGAAAAAT-3' and reverse, 5'-TTCAAGGTCAAGACGTGCCA-3'; and for normalization, GAPDH forward, 5'-GCATCTTCTTGTGCAGTGCC-3' and reverse, 5'-TACGGCCAAATCCGTTCACA-3'.
The relative mRNA expression levels of vimentin and α-SMA were quantified with respect to the expression of the housekeeping gene, GAPDH.
6. Immunofluorescence
To investigate the influence of silymarin on the expression of vimentin and α-SMA in NRK-49F cells under TGF-β1 stimulation, we conducted immunofluorescence staining following standardized protocols. Briefly, NRK-49F cells were cultured on glass coverslips, permitting adequate attachment, and spread. These cells were then pretreated with silymarin for 16 hours, followed by a 6-hour stimulation with TGF-β1. Subsequent to this treatment, cells were fixed using 4% paraformaldehyde for 15 minutes at room temperature, and then permeabilized using 0.1% Triton X-100 for an additional 10 minutes. Non-specific binding sites were blocked with 1% bovine serum albumin (BSA) in phosphate-buffered saline (PBS) for an hour at room temperature. Cells were then incubated overnight at 4℃ with primary antibodies targeting vimentin and α-SMA, each diluted at a 1:500 ratio in the blocking buffer. Following the incubation with primary antibodies, cells were washed 3 times with PBS, and then incubated with corresponding Alexa 488-conjugated secondary antibodies for 1 hour at room temperature, with measures taken to shield from light. Following this, coverslips were mounted onto microscope slides using an anti-fade mounting medium with DAPI (4',6-diamidino-2-phenylindole). The prepared samples were examined under a fluorescence microscope, with images captured for further analysis. To ensure the reliability of our findings, all staining procedures were conducted in triplicate. Images were captured using a confocal microscope (LSM710) at the Three-Dimensional Immune System Imaging Core Facility of Ajou University.
Results
1. Cytotoxicity assessment reveals minimal effects of silymarin
To evaluate the cytotoxicity of silymarin (3,5,7-trihydroxy-2-[3-(4-hydroxy-3-methoxyphenyl)-2-(hydroxymethyl)-2,3-dihydro-1,4-benzodioxin-6-yl]-3,4-dihydro-2H-1-benzopyran-4-one), a flavonolignan compound with known cell-protective properties (Fig. 1A), we treated NRK-49F cells with various concentrations of silymarin and measured cell viability. The EZ-cytotox assay revealed an IC50 value of 135.8 μM, indicating minimal cytotoxic effects of silymarin (Fig. 1B). Consequently, subsequent experiments were conducted using a maximum concentration of 50 μM.
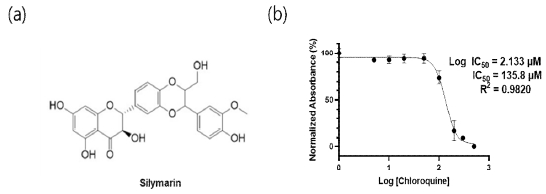
The low cytotoxic effect of silymarin on NRK-49F cells. (a) The chemical structure of the silymarin (b) NRK-49F cells were exposed to various concentrations of silymarin (ranging from 1µM to 500µM) for a duration of 72 hours, followed by an assessment of cell viability employing the EZ-cytox assay. IC50 = 135.8µM
2. Inhibition of fibroblast-to-myofibroblast transition (FMT) by silymarin in NRK-49F cells
To investigate the potential inhibitory effect of silymarin on TGF-β1-induced FMT, NRK-49F cells were treated with silymarin at concentrations of 20 μM and 50 μM for 72 hours following TGF-β1 stimulation. As shown in Fig. 2(a), TGF-β1 treatment resulted in an upregulation of α-SMA and vimentin, markers associated with myofibroblasts. However, silymarin effectively suppressed the TGF-β1-induced increase in α-SMA and vimentin expression, suggesting its inhibitory potential in modulating key genes involved in initiating FMT. Furthermore, mRNA expression levels of α-SMA and vimentin were analyzed using RT-PCR to further assess the inhibitory influence of silymarin on FMT. As depicted in Fig. 2(b), silymarin concentrations exceeding 20 μM significantly attenuated the TGF-β1-mediated upregulation of α-SMA and vimentin mRNA expressions in NRK-49F cells. These findings provide additional evidence for the suppressive effect of silymarin on the fibroblast-to-myofibroblast transition process.
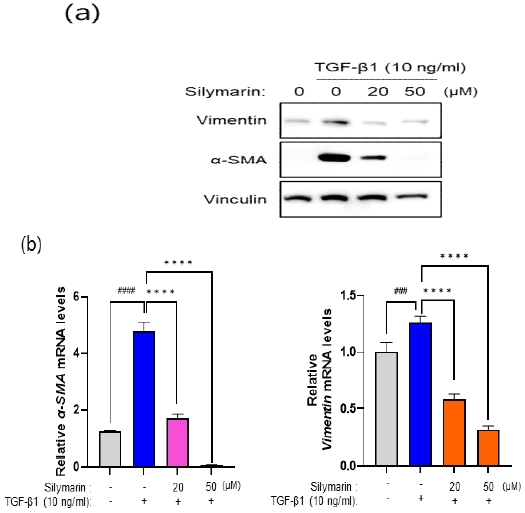
Silymarin suppresses the TGF-β1-induced FMT in NRK-49F Cells. (a) NRK-49F cells were co-treated with silymarin (20, 50μM) and TGF-β (10ng/ml) for 72 hours. Subsequently, cell lysates were obtained, and the protein expression levels of FMT markers, α-SMA and vimentin, were analyzed by western blotting. Silymarin significantly suppressed the expression of both proteins. (b) NRK-49F cells were pre-treated with silymarin (20, 50 μM) for 16 hours, followed by a 4-hour stimulation with TGF-β (10 ng/ml). After treatment, RNA was isolated from the cells and converted into cDNA. Real-time PCR analysis was performed using specific primers for vimentin and α-SMA. The data revealed that silymarin markedly reduced the mRNA expression of vimentin and α-SMA at concentrations exceeding 20 μM. Values are presented as the mean ± SD. The statistical analysis of a-SMA and vimentin mRNA expression was performed using one-way ANOVA, yielding F values of 133.6 and 179.8, respectively, with p values less than 0.0001 for both comparisons. ####p < 0.0001 , ###p < 0.001indicates significant differences from the control group (1st column) and **** p < 0.0001 indicate significant difference from the positive control (2nd column).
3. Fluorescence microscopy analysis reveals the inhibitory role of silymarin on FMT markers
To investigate the inhibitory role of silymarin on FMT, we employed fluorescence microscopy to analyze the expression of α-SMA and vimentin in NRK-49F cells. As illustrated in Fig. 3, TGF-β1 treatment induced pronounced vimentin expression predominantly localized sub-membranously, along with upregulated α-SMA expression in both nuclear and cytoplasmic compartments. Conversely, a 16-hour pre-incubation with silymarin notably attenuated the expression of these proteins, particularly at concentrations exceeding 20 μM, further supporting its inhibitory role in modulating FMT.
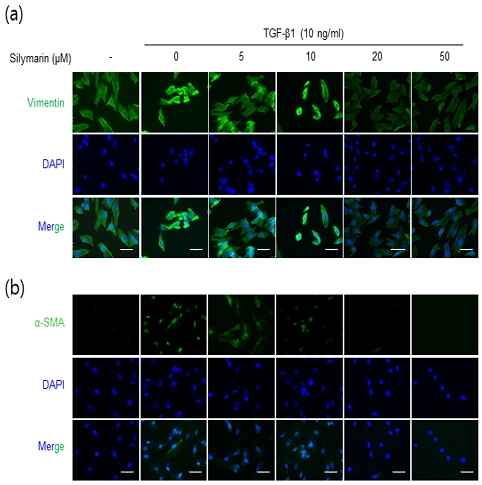
Immunofluorescence evaluation of silymarin’s influence on FMT markers α-SMA and vimentin expression in NRK-49F Cells. NRK-49F cells were pretreated with silymarin at various concentrations for 16 hours followed by a 6-hour treatment with TGF-β (10 ng/ml). Fluorescence microscopy was used to visualize the expression patterns of vimentin (a) and α-SMA (b) within the cells. The data demonstrate a significant reduction in TGF-β-induced vimentin and α-SMA expression at silymarin concentrations exceeding 20 μM. The bar scale at the bottom represents 50 μm.
4. Modulation of signaling pathways by silymarin in the suppression of vimentin and α-SMA expression
To elucidate the molecular mechanisms underlying the suppressive effects of silymarin on vimentin and α-SMA expression, we conducted an analysis of the phosphorylation status of key signaling proteins, including Smad-2, p38, JNK, and mTOR. NRK-49F cells were preincubated with various concentrations of silymarin for 16 hours, followed by exposure to TGF-β1 for 1 hour. Subsequently, cellular lysates were harvested, and western blot analysis was performed to assess the phosphorylation levels of the signaling proteins.
While silymarin did not exert an inhibitory effect on the phosphorylation of Smad2, which is a key component of the canonical signaling pathway of TGF-β (Fig. 4(a)), it exhibited inhibitory effects on p38 and JNK signaling pathways, particularly at higher concentrations (Fig. 4(c)). This suggests that silymarin may modulate alternative signaling pathways involved in TGF-β-mediated fibrotic processes. Notably, silymarin effectively suppressed the phosphorylation of p70 S6 Kinase (p70 S6K) (Fig. 4b). These findings provide compelling evidence that silymarin exerts inhibitory effects on the expression of vimentin and α-SMA through the regulation of mTOR signaling pathways implicated in the process of FMT.
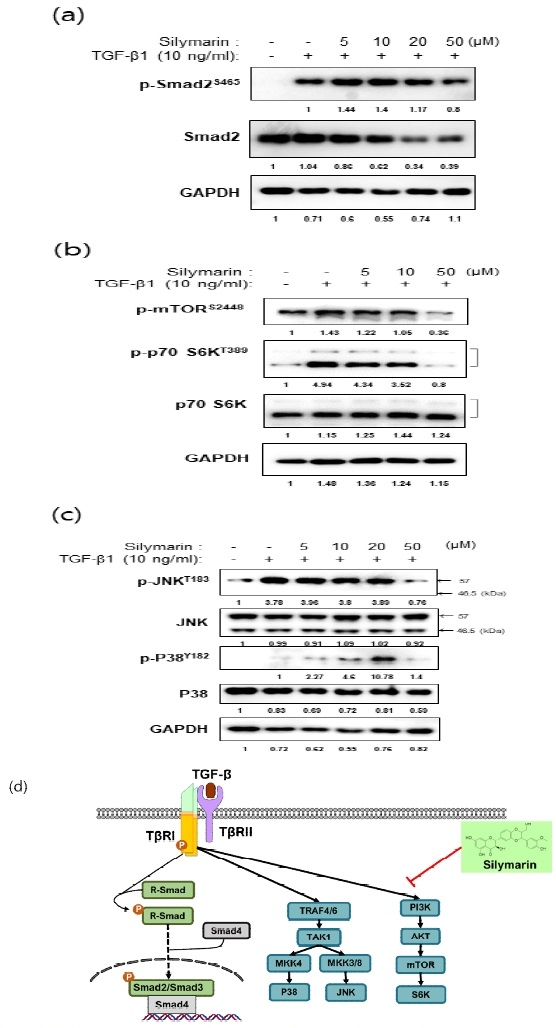
Effects of silymarin on cell signaling pathways in TGF-β-stimulated NRK-49F cells. NRK-49F cells were pretreated with different concentrations of silymarin for 16 hours, followed by stimulation with TGF-β for 1 hour. Subsequently, the phosphorylation status of intracellular signaling proteins was examined. Notably, silymarin demonstrated significant inhibition of the mTOR pathway (b) compared to the Smad pathway (a), or the JNK and p38 pathways (c). (d) Graphical representation illustrating the specific inhibitory effect of silymarin on the mTOR pathway within the TGF-β signaling cascade.
Discussion
Renal fibrosis (RF) is a major contributor to the progression of chronic kidney disease (CKD) and is associated with significant morbidity and mortality2,4). Currently, effective therapeutic strategies for RF are limited, underscoring the urgent need for novel treatment approaches16). The findings of this study demonstrate that silymarin effectively inhibits fibroblast-to-myofibroblast transition (FMT), a key process involved in RF17,18). By targeting FMT, silymarin holds promise as a therapeutic intervention to mitigate fibrotic processes and potentially halt or slow down the progression of renal fibrosis.
In this study, we investigated the potential inhibitory effects of silymarin on FMT in NRK-49F cells, the renal interstitial fibroblasts. Our results demonstrate the effectiveness of silymarin in suppressing FMT, highlighting its potential as a therapeutic agent for the management of fibrotic diseases, including RF.
First, we assessed the cytotoxicity of silymarin in NRK-49F cells. The EZ-cytotox assay revealed minimal cytotoxic effects, as indicated by the IC50 value of 135.8 μM, suggesting that silymarin has a low cytotoxic profile (Fig. 1b). Accordingly, subsequent experiments were conducted using concentrations up to 50 μM, which ensured a safe and effective dosage range.
We investigated the inhibitory effect of silymarin on FMT in NRK-49F cells. Exposure of the cells to TGF-β1, a potent inducer of FMT, led to an upregulation of vimentin and α-SMA, markers associated with myofibroblast activation. The treatment with silymarin (20, 50 μM) resulted in a significant downregulation of vimentin and α-SMA expression (Fig. 2a). These findings indicate that silymarin effectively suppresses the conversion of fibroblasts into myofibroblasts, suggesting its potential in mitigating fibrotic processes, such as RF.
Additionally, explore the inhibitory influence of silymarin on FMT, we analyzed the mRNA expression levels of α-SMA and vimentin using RT-PCR. Our results demonstrated that silymarin concentrations exceeding 20 μM significantly attenuated the TGF-β1-induced upregulation of α-SMA and vimentin mRNA expressions in NRK-49F cells (Fig. 2b). This further confirms the ability of silymarin to modulate the transcriptional regulation of FMT markers, reinforcing its potential therapeutic value in fibrotic diseases.
To further, we employed fluorescence microscopy to visualize the expression of α-SMA and vimentin in NRK-49F cells treated with silymarin and TGF-β1. TGF-β1 stimulation resulted in pronounced vimentin expression, predominantly localized sub-membranously, and upregulated α-SMA expression in both nuclear and cytoplasmic compartments. However, pre-incubation with silymarin for 16 hours notably attenuated the expression of these proteins, particularly at concentrations exceeding 20 μM (Fig. 3a, b). These findings provide visual evidence of silymarin's ability to counteract FMT processes, further supporting its potential as a therapeutic intervention for fibrotic conditions.
To elucidate the molecular mechanism underlying the inhibitory effect of silymarin, we investigated the activation of key proteins in the signaling pathway involved in fibroblast-to-myofibroblast transition (FMT) regulation. Specifically, we assessed the phosphorylation levels of Smad2, JNK, p38 MAPK, and mTOR in NRK-49F cells treated with silymarin and TGF-β1. Our results demonstrated that silymarin did not inhibit the phosphorylation of Smad2 (Fig. 4a). However, at a concentration of 50 μM, silymarin exhibited significant inhibition of JNK and p38 phosphorylation (Fig. 4c). Notably, silymarin prominently suppressed the phosphorylation of p70 S6 kinase (p70 S6K) (Fig. 4b). These findings provide compelling evidence supporting the notion that silymarin's inhibitory effects on TGF-β1-induced FMT primarily involve the modulation of the non-canonical mTOR signaling pathway.
To date, extensive research has been conducted on the anti-fibrotic effects of polyphenols, which are known for their anti-inflammatory and antioxidant properties. These polyphenols have demonstrated the ability to regulate immune cell proliferation and mitigate chronic inflammation 19). Consequently, they have emerged as promising candidates for targeting RF, and preliminary studies have provided valuable insights.
Curcumin, a constituent of Curcumaelongae Rhizoma, has garnered attention for its inhibitory effects on RF. Studies have shown that curcumin exerts its anti-fibrotic effects by suppressing the activity of the NLRP3 inflammasome and the PI3K/mTOR signaling pathway. These mechanisms contribute to the inhibition of fibrotic processes in the kidney20). Resveratrol, extracted from grapes and peanuts, has also demonstrated potential in inhibiting RF. Its anti-fibrotic properties are attributed to its ability to inhibit myofibroblastic phenotype21). Epigallocatechin gallate (EGCG), a prominent polyphenolic component found in green tea, has been investigated for its inhibitory effects on RF. Studies have highlighted its ability to counteract excessive ECM deposition in renal cells22), induce fibroblast apoptosis23), and inhibit the TGF-β/Smad signaling pathway24). These mechanisms collectively contribute to the suppression of fibrotic processes in the kidney. Salvianolic acid A derived from Radix Salviae has also shown promising nephroprotective effects by inhibiting the expression of TGF-β1 and α-SMA in renal cells. By modulating these key markers of fibroblast activation, salvianolic acid A has the potential to mitigate RF25).
The accumulating evidence on the anti-fibrotic effects of polyphenols, including curcumin, resveratrol, EGCG, and salvianolic acid A, underscores their potential therapeutic value in RF. These polyphenols represent promising avenues for the development of novel therapeutic strategies for RF and warrant further attention and exploration in future research endeavors.
Silymarin, complex mixture of flavonolignans derived from the milk thistle plant (Silybum marianum), possesses a rich traditional history in the realm of traditional medicine, owing to its well-documented antioxidant, anti-inflammatory, and hepatoprotective properties19-21). Expanding the application of silymarin to the field of RF introduces novel possibilities for therapeutic interventions. Nevertheless, it is imperative to acknowledge that further investigations are warranted to comprehensively establish the clinical relevance and safety of silymarin in the context of RF. Robust preclinical studies, employing suitable animal models, as well as well-designed clinical trials, are essential to substantiate the efficacy, determine optimal dosage regimens, and ascertain the long-term safety profiles of silymarin as a viable treatment option for RF. In conclusion, the findings of this study shed light on the clinical significance of targeting FMT as a therapeutic strategy for RF. The observed inhibitory effects of silymarin on FMT markers, coupled with its potential as a safe and accessible therapeutic agent, hold promise for the development of novel therapies for RF and the management of CDK.
Acknowledgments
This work was supported by a 2-Year Research Grant of Pusan National University.
References
-
Kovesdy CP. Epidemiology of chronic kidney disease: an update 2022. Kidney Int Suppl (2011). 2022;12(1):7-11.
[https://doi.org/10.1016/j.kisu.2021.11.003]
-
Huang R, Fu P, Ma L. Kidney fibrosis: from mechanisms to therapeutic medicines. Signal Transduct Target Ther. 2023;8(1):129.
[https://doi.org/10.1038/s41392-023-01379-7]
-
Humphreys BD. Mechanisms of Renal Fibrosis. Annual review of physiology. 2018;80:309-26.
[https://doi.org/10.1146/annurev-physiol-022516-034227]
-
Panizo S, Martinez-Arias L, Alonso-Montes C, Cannata P, Martin-Carro B, Fernandez-Martin JL, et al. Fibrosis in Chronic Kidney Disease: Pathogenesis and Consequences. International journal of molecular sciences. 2021;22(1).
[https://doi.org/10.3390/ijms22010408]
-
Liu F, Zhuang S. New Therapies for the Treatment of Renal Fibrosis. Adv Exp Med Biol. 2019;1165:625-59.
[https://doi.org/10.1007/978-981-13-8871-2_31]
-
Yuan Q, Tan RJ, Liu YH. Myofibroblast in Kidney Fibrosis: Origin, Activation, and Regulation. Renal Fibrosis: Mechanisms and Therapies. 2019;1165:253-83.
[https://doi.org/10.1007/978-981-13-8871-2_12]
-
Wynn TA, Ramalingam TR. Mechanisms of fibrosis: therapeutic translation for fibrotic disease. Nat Med. 2012;18(7):1028-40.
[https://doi.org/10.1038/nm.2807]
-
Meng XM, Nikolic-Paterson DJ, Lan HY. TGF-beta: the master regulator of fibrosis. Nature reviews Nephrology. 2016;12(6):325-38.
[https://doi.org/10.1038/nrneph.2016.48]
-
Meng XM, Tang PM, Li J, Lan HY. TGF-beta/Smad signaling in renal fibrosis. Frontiers in physiology. 2015;6:82.
[https://doi.org/10.3389/fphys.2015.00082]
-
Hackett ES, Twedt DC, Gustafson DL. Milk thistle and its derivative compounds: a review of opportunities for treatment of liver disease. J Vet Intern Med. 2013;27(1):10-6.
[https://doi.org/10.1111/jvim.12002]
-
Surai PF. Silymarin as a Natural Antioxidant: An Overview of the Current Evidence and Perspectives. Antioxidants (Basel). 2015;4(1):204-47.
[https://doi.org/10.3390/antiox4010204]
-
Chlopcikova S, Psotova J, Miketova P, Simanek V. Chemoprotective effect of plant phenolics against anthracycline-induced toxicity on rat cardiomyocytes. Part I. Silymarin and its flavonolignans. Phytother Res. 2004;18(2):107-10.
[https://doi.org/10.1002/ptr.1415]
-
Hou YC, Liou KT, Chern CM, Wang YH, Liao JF, Chang SO, et al. Preventive effect of silymarin in cerebral ischemia-reperfusion-induced brain injury in rats possibly through impairing NF-kappa B and STAT-1 activation. Phytomedicine : international journal of phytotherapy and phytopharmacology. 2010;17(12):963-73.
[https://doi.org/10.1016/j.phymed.2010.03.012]
-
Katiyar SK. Treatment of silymarin, a plant flavonoid, prevents ultraviolet light-induced immune suppression and oxidative stress in mouse skin. Int J Oncol. 2002;21(6):1213-22.
[https://doi.org/10.3892/ijo.21.6.1213]
-
Chen Y, Chen L, Yang TW. Silymarin nanoliposomes attenuate renal injury on diabetic nephropathy rats via co-suppressing TGF-beta/Smad and JAK2/STAT3/SOCS1 pathway. Life sciences. 2021;271.
[https://doi.org/10.1016/j.lfs.2021.119197]
-
Yan H, Xu J, Xu Z, Yang B, Luo P, He Q. Defining therapeutic targets for renal fibrosis: Exploiting the biology of pathogenesis. Biomed Pharmacother. 2021;143:112115.
[https://doi.org/10.1016/j.biopha.2021.112115]
-
Kuppe C, Ibrahim MM, Kranz J, Zhang X, Ziegler S, Perales-Paton J, et al. Decoding myofibroblast origins in human kidney fibrosis. Nature. 2021;589(7841):281-6.
[https://doi.org/10.1038/s41586-020-2941-1]
-
Zhao Q, Shao T, Zhu Y, Zong G, Zhang J, Tang S, et al. An MRTF-A-ZEB1-IRF9 axis contributes to fibroblast-myofibroblast transition and renal fibrosis. Experimental & molecular medicine. 2023.
[https://doi.org/10.1038/s12276-023-00990-6]
-
Yahfoufi N, Alsadi N, Jambi M, Matar C. The Immunomodulatory and Anti-Inflammatory Role of Polyphenols. Nutrients. 2018;10(11).
[https://doi.org/10.3390/nu10111618]
-
Lu M, Li H, Liu W, Zhang X, Li L, Zhou H. Curcumin attenuates renal interstitial fibrosis by regulating autophagy and retaining mitochondrial function in unilateral ureteral obstruction rats. Basic Clin Pharmacol Toxicol. 2021;128(4):594-604.
[https://doi.org/10.1111/bcpt.13550]
-
Zhang X, Lu H, Xie S, Wu C, Guo Y, Xiao Y, et al. Resveratrol suppresses the myofibroblastic phenotype and fibrosis formation in kidneys via proliferation-related signalling pathways. British journal of pharmacology. 2019;176(24):4745-59.
[https://doi.org/10.1111/bph.14842]
-
Chen J, Du L, Li J, Song H. Epigallocatechin-3-gallate attenuates cadmium-induced chronic renal injury and fibrosis. Food Chem Toxicol. 2016;96:70-8.
[https://doi.org/10.1016/j.fct.2016.07.030]
-
Luo D, Xu JP, Chen XJ, Zhu X, Liu S, Li J, et al. (-)-Epigallocatechin-3-gallate (EGCG) attenuates salt-induced hypertension and renal injury in Dahl salt-sensitive rats (vol 10, 4783, 2020). Scientific reports. 2020;10(1).
[https://doi.org/10.1038/s41598-020-61794-6]
-
Zhu QQ, Yang XY, Zhang XJ, Yu CJ, Pang QQ, Huang YW, et al. EGCG targeting Notch to attenuate renal fibrosis via inhibition of TGFbeta/Smad3 signaling pathway activation in streptozotocin-induced diabetic mice. Food Funct. 2020;11(11):9686-95.
[https://doi.org/10.1039/D0FO01542C]
-
Zhang HF, Wang YL, Gao C, Gu YT, Huang J, Wang JH, et al. Salvianolic acid A attenuates kidney injury and inflammation by inhibiting NF-kappaB and p38 MAPK signaling pathways in 5/6 nephrectomized rats. Acta Pharmacol Sin. 2018;39(12):1855-64.
[https://doi.org/10.1038/s41401-018-0026-6]
-
Saller R, Meier R, Brignoli R. The use of silymarin in the treatment of liver diseases. Drugs. 2001;61(14):2035-63.
[https://doi.org/10.2165/00003495-200161140-00003]
-
Gazak R, Walterova D, Kren V. Silybin and silymarin--new and emerging applications in medicine. Current medicinal chemistry. 2007;14(3):315-38.
[https://doi.org/10.2174/092986707779941159]
- Kidd PM. Bioavailability and activity of phytosome complexes from botanical polyphenols: the silymarin, curcumin, green tea, and grape seed extracts. Altern Med Rev. 2009;14(3):226-46.