
Echinacea purpurea extract inhibits LPS-induced inflammatory response by interfering with TLR4-mediated NF-κB and MAPKs signaling pathways
Ⓒ The Society of Pathology in Korean Medicine, The Physiological Society of Korean Medicine
Abstract
Echinacea purpurea (Asteraceae family) is widely used in the European countries and the United States due to its proven immune enhancement and anti-inflammatory effects. Echinacea purpurea has been reported prevent and treat upper respiratory tract infections and common cold, but the underlying molecular mechanisms are not well understood. In the present study, we examined the anti-inflammatory effects and molecular mechanisms of Echinacea purpurea (EP) extract using lipopolysaccharide (LPS)-stimulated signal pathways in RAW264.7 cells. Our results suggest that EP extract exerts anti-inflammatory effects by down-regulating the expression of LPS-induced toll-like receptor 4 (TLR4), subsequently inhibiting the activation of nuclear factor (NF)-κB and mitogen-activated protein kinase (MAPK) signaling pathways and suppression of the release of pro-inflammatory cytokines. These results suggest that EP extract is a potential therapeutic agent for inflammatory diseases.
Keywords:
Echinacea purpurea extract, inflammatory response, TLR4, NF-κB, MAPKIntroduction
The innate immune system is the host’s first line of defense during infection and plays an important role in the early recognition and subsequent induction of inflammatory responses to invading pathogens.1) The innate immune system is primarily mediated by macrophages and dendritic cells, and the pattern recognition receptors (PRRs) of these immune cells bind to structures called pathogen-associated molecular patterns (PAMPs) of pathogens.2-6)
Macrophages, the major pro-inflammatory cells, are activated by bacterial products such as lipopolysaccharide (LPS) to produce various inflammatory cytokines including interleukin-2 (IL-2), IL-6, and IL-1β, tumor necrosis factor-α (TNF-α), interferon-γ (IFN-γ), and other inflammatory mediators like as nitric oxide (NO).7,8) Therefore, downregulating the expression of these pro-inflammatory mediators and therefore, cytokines, could ameliorate the inflammatory diseases.9)
Lipopolysaccharide, a common PAMP, is a main composition of the outer membrane of Gram-negative bacteria and initiates a signaling cascade through interaction with the toll-like receptor 4 (TLR4).10) When TLR4 is activated by LPS, protein complexes like as TNF receptor-associated factor 6 (TRAF6) are recruited. Then, association of TRAF6 and transforming growth factor-β (TGF-β)-activated kinase 1 (TAK1) activates the downstream signaling nuclear factor (NF)-κB, and mitogen-activated protein kinases (MAPKs).11)
NF-κB exists in an inactive form in the cytoplasm and binds to the inhibitory protein IκBα. Upon stimulation of the pro-inflammatory signals, IκBα is phosphorylated by IκB kinase (IKK).12) After phosphorylation of IκB, the degraded and released NF-κB translocate to the nucleus and binds to the κB-binding site in the promoter region of the target genes and induce the transcription of pro-inflammatory mediators.13)
The MAPKs, including extracellular signal regulated kinase (ERK), c-jun N-terminal kinase (JNK), and p38, respond to the extra-and intra-cellular stimuli and regulate immune responses, including pro-inflammatory cytokine production, cell proliferation, differentiation, and survival/apoptosis.14,15)
Echinacea purpurea, a flower in the Asteraceae family, is widely known for its immunomodulatory effects and it is widely used in the United States and the European countries because of its proven immune enhancement and anti-inflammatory effects. Especially, Echinacea purpurea has been used extensively for the prevention and treatment of upper respiratory tract infections and common cold, but the underlying molecular mechanisms are not well understood.16-20) Therefore, the present study aimed to demonstrate the anti-inflammatory effects and the underlying molecular mechanisms of Echinacea purpurea (EP) extract in LPS-induced RAW264.7 cells.
Materials and Methods
1. Sample preparation
Echinacea purpurea (L.) Moench aerial parts were extracted using 60% EtOH. The resulting solution was concentrated and dried to yield EP extract. The finished formulation was standardized to approximately 2% chicoric acid. This ingredient is produced by Evear Extraction (Féline, France). Then the EP extract was dissolved in dimethyl sulfoxide (DMSO) for in vitro studies.
2. Chemicals and reagents
Mouse RAW264.7 cells were provided by American Type Culture Collection (ATCC; Rockville, MD, USA). Dulbecco’s modified Eagle’s medium (DMEM), fetal bovine serum (FBS), and penicillin-streptomycin were provided by Gibco BRL (Grand Island, NY, USA). LPS (Escherichia coli O111:B4) was provided from Sigma-Aldrich Co (St. Louis, MO, USA). iNOS was obtained from the Invitrogen Life Technologies (Carlsbad, CA, USA). IL-2 and IL-10 were purchased from the Santa Cruz Biotechnology (Santa Cruz, CA, USA). IL-6, IL-1β, IFN-γ, and TNF-α were purchased from the Abcam (Cambridge, UK). p-IKKαβ, p-IκBα, p-NF-κB p65, NF-κB p65, p-ERK, ERK, p-p38, p38, TLR4, TRAF6, p-TAK1, TAK1, and β-actin were purchased from the Cell Signaling Technology, Inc (Danvers, MA, USA). HRP-conjugated goat anti-rabbit and goat anti-mouse antibodies were purchased from GenDEPOT (Barker, TX, USA).
3. Cell culture
RAW264.7 cells were maintained in DMEM supplemented with 10% heat-inactivated FBS, 100 units/mL penicillin, and 100 μg/mL streptomycin and cultured under a humidified atmosphere at 37 ℃ and 5% CO2.
4. Cell viability assay
Cell viability was assessed by the 3-[4, 5-dimethylthiazol-2-yl]-2,5 diphenyl tetrazolium bromide (MTT) assay. RAW264.7 cells were treated with EP extract at concentration of 12.5, 25, or 50 μg/mL for 24 h. MTT solution (5 mg/mL) was added to each well, and the cells were incubated for 3 h at 37 ℃. The supernatant was removed from each well, and formazan crystals were resolved with DMSO. Cell viability was determined from the absorbance read with a microplate reader (Tecan, Männedorf, Switzerland) at a wavelength of 570 nm.
5. Measurement of nitric oxide (NO) production
NO concentrations in cell culture supernatants were measured using Griess reagent according to the manufacturer’s instructions (Promega, Madison, WI, USA). RAW264.7 cells were stimulated with LPS (100 ng/mL) and EP extract (12.5, 25, or 50 μg/mL) for 24 h, then the cell culture supernatants were harvested and briefly centrifuged. Next 50 μL of the cell culture supernatants was mixed with 100 μL of Griess reagent and reacted for 10 min at room temperature in the dark. Absorbance was determined using a microplate reader at a wavelength of 570 nm.
6. Protein extraction and western blot analysis
Western blot analysis was executed by lysing cells in RIPA buffer (DYNE Bio, Seongnam, Korea) containing a protease inhibitor cocktail tablet (Roche, Mannheim, Germany). BCA protein assay kit (Thermo, Waltham, USA) was used to measure the total protein concentration of each lysate. Protein was electrophoresed on SDS-PAGE and moved onto membranes (Millipore Corp., Bedford, MA, USA). For blocking, the membranes were kept for 1 h at 23 ℃ and then reacted with the primary antibodies (1:1000) against iNOS, IL-2, IL-6, IL-10, IL-1β, IFN-γ, TNF-α, p-IKKαβ, p-IκBα, p-NF-κB p65, NF-κB p65, p-ERK, ERK, p-p38, p38, TLR4, TRAF6, p-TAK1, TAK1, or β-actin at 4 ℃ for overnight. The incubated membranes were washed and further reacted with goat anti-rabbit IgG(H+L)-HRP or goat anti-mouse IgG(H+L)-HRP secondary antibodies (1:10000) at 23 ℃ for 1 h. The membranes were processed using ECL solution (Atto, Tokyo, Japan) for detection and the band intensity was analyzed using Image-Pro Plus (Media Cybernetics, Inc., USA). The β-actin was used as a control for normalization. Bands on the membranes were quantified with the ImageJ program (developed at the NIH).
7. Statistical analysis
All data are presented as the mean ± standard deviation. Groups were compared using the student’s t-test and one-way analysis of variance, as applicable. Statistical analyses were performed using an Origin 7 software (Microcal Software, Northampton, MA, USA). P< 0.05 and P< 0.01 were considered to be statistically significant.
Results
1. Effects of EP extract on cell viability
To confirm the cytotoxicity effects of EP extract on RAW264.7 cells, we exposed the cells to various concentrations of EP extract (12.5, 25, or 50 μg/mL) for 24 h. As shown in Fig. 1A, EP extract was not cytotoxic up to 50 μg/mL. Therefore, we selected 12.5, 25, or 50 μg/mL EP extract concentrations for subsequent in vitro experiments.
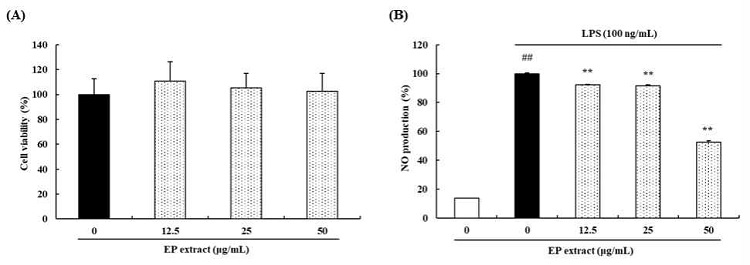
Effects of EP extract on cell viability and NO production. (A) RAW264.7 cells were treated with various concentrations of EP extract (12.5, 25, or 50 μg/mL) for 24 h. The cell viability was evaluated using the MTT assay. (B) RAW264.7 cells were stimulated with LPS (100 ng/mL) in the absence or presence of EP extract (12.5, 25, or 50 μg/mL) for 24 h. NO production levels in the culture supernatants were measured using Griess reagent. The data presented are the mean ± standard deviation of three independent experiments and differences between the mean values. ##P< 0.01 vs. Control group; **P< 0.01 vs. LPS group.
2. Effects of EP extract on NO production
We examine the possibility of EP extract to inhibit LPS-induced NO production. Based on our findings, LPS 100 ng/mL treatment increased the NO production. On the other hand, treatment with EP extract at 12.5, 25, or 50 μg/mL decreased the LPS-mediated NO production by 7.87%, 8.49%, and 47.50%, respectively(Fig. 1B).
3. Effects of EP extract on inflammatory cytokines in LPS-stimulated RAW264.7 cells
iNOS plays important role in the synthesis of NO. Thus, we investigated whether the EP extract influenced the expression of iNOS in RAW264.7 cells. Treatment with EP extract suppressed the expression of iNOS (18.46%) in LPS-treated RAW264.7 cells (Fig. 2).
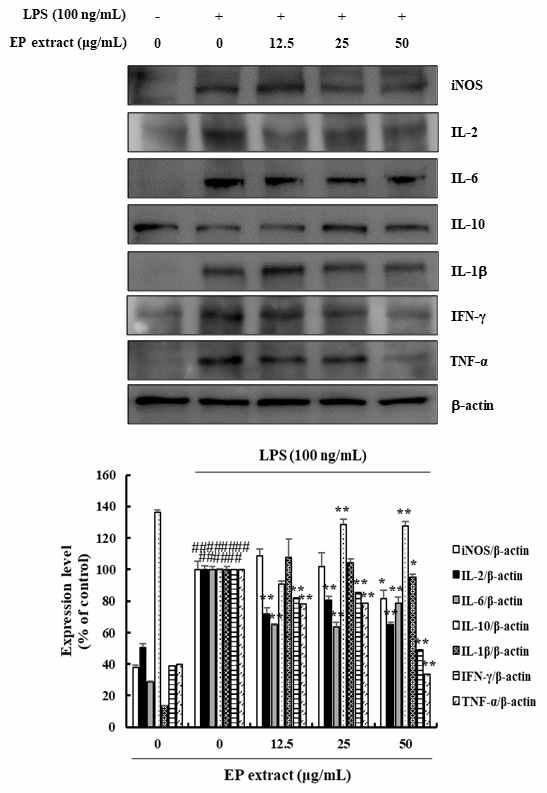
Effects of EP extract on inflammatory cytokines in LPS-stimulated RAW264.7 cells. RAW264.7 cells were stimulated with LPS (100 ng/mL) in the absence or presence of EP extract (12.5, 25, or 50 μg/mL) for 24 h. Protein expressions were analyzed by western blot with specific antibodies. β-actin was used as a control. The data presented are the mean ± standard deviation of three independent experiments and differences between the mean values. ##P< 0.01 vs. Control group; *P< 0.05, **P< 0.01 vs. LPS group.
To analyze the potential anti-inflammatory effects of EP extract, we investigated whether EP extract influenced the expression of pro-inflammatory cytokines in LPS-stimulated RAW264.7 cells. The protein expression of IL-2, IL-6, IL-1β, IFN-γ, and TNF-α were evaluated using western blotting. The results showed that EP extract suppressed IL-2 (19.53-35.07%), IL-6 (21.35-36.50%), IL-1β (4.80%), IFN-γ (14.81-50.91%), and TNF-α (21.34-66.30%) protein expression in LPS-stimulated RAW264.7 cells (Fig. 2). We also confirmed the presence of anti-inflammatory cytokine such as IL-10 in LPS-stimulated RAW264.7 cells. IL-10 was significantly increased (27.41-28.55%) in EP extract treated RAW264.7 cells when compared with LPS-stimulated cells (Fig. 2). These results clearly demonstrated the anti-inflammatory activity in LPS-induced inflammation in RAW264.7 cells.
4. Effects of EP extract on NF-κB signaling pathway in LPS-stimulated RAW264.7 cells
To examine whether the inhibition of inflammatory response by EP extract is mediated through the NF-κB pathway, we examined the effects of EP extract treatment on LPS-induced phosphorylation of IKKαβ, IκBα, and NF-κB p65 were determined by western blotting. The results showed that EP extract significantly inhibited LPS-induced phosphorylation of IKKαβ, IκBα, and NF-κB p65 in the range of 23.06-30.31%, 9.87-31.03%, and 5.88-30.94%, respectively (Fig. 3).
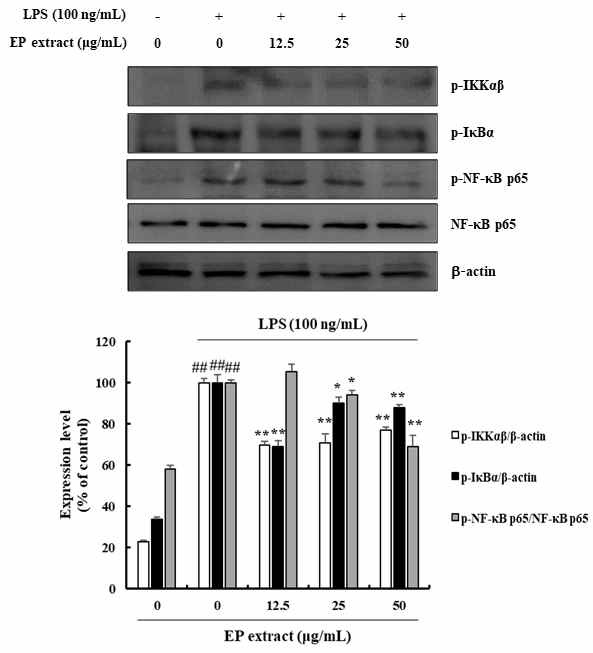
Effects of EP extract on NF-κB signaling pathway in LPS-stimulated RAW264.7 cells. RAW264.7 cells were treated with LPS (100 ng/mL) in the absence or presence of EP extract (12.5, 25, or 50 μg/mL) for 24 h. Protein expressions were analyzed by western blot with specific antibodies. β-actin or NF-κB p65 were used as controls. The data presented are the mean ± standard deviation of three independent experiments and differences between the mean values. ##P< 0.01 vs. Control group; *P< 0.05, **P< 0.01 vs. LPS group.
5. Effects of EP extract on MAPK signaling pathway in LPS-stimulated RAW264.7 cells
MAPK family plays a critical role in the production of inflammatory mediators upon activation by LPS. We thus investigated the effect of EP extract on LPS-induced MAPKs phosphorylation and observed that LPS-induced ERK phosphorylation (0.10-16.04%) and p38 phosphorylation (0.95-13.07%), which were strongly suppressed by EP extract treatment in RAW264.7 cells (Fig. 4).
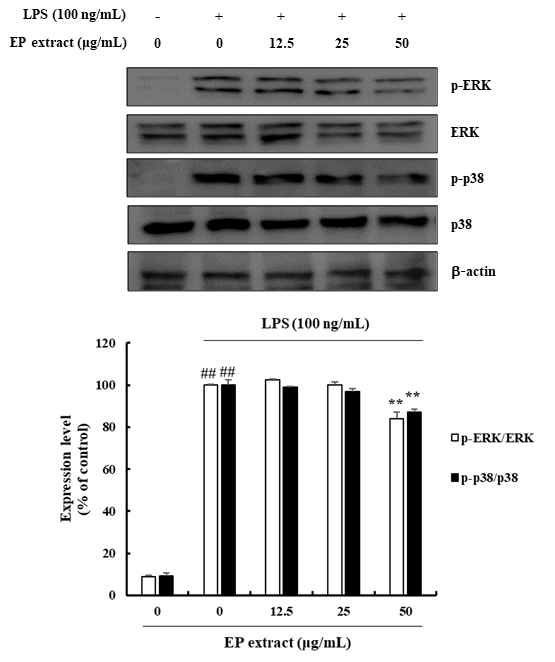
Effects of EP extract on MAPK signaling pathway in LPS-stimulated RAW264.7 cells. RAW264.7 cells were stimulated with LPS (100 ng/mL) in the absence or presence of EP extract (12.5, 25, or 50 μg/mL) for 24 h. Protein expressions were analyzed by western blot with specific antibodies. ERK or p38 were used as controls. The data presented are the mean ± standard deviation of three independent experiments and differences between mean values. ##P< 0.01 vs. Control group; **P< 0.01 vs. LPS group.
6. Effects of EP extract on TLR4 in LPS-stimulated RAW264.7 cells
To understand the mechanism through which EP extract-mediated inflammatory response are regulated, we investigated the expression of TLR4 in LPS-induced RAW264.7 cells treated with EP extract. The expression of TLR4 and TRAF6 and the phosphorylation of TAK1 by LPS stimulation in RAW264.7 cells were significantly inhibited by EP extract in the range of 29.60-81.59%, 18.00-30.28%, and 19.78-41.21%, respectively (Fig. 5). Thus, EP extract suppresses LPS-induced inflammation via the TLR4-mediated NF-κB and MAPKs signaling pathways.
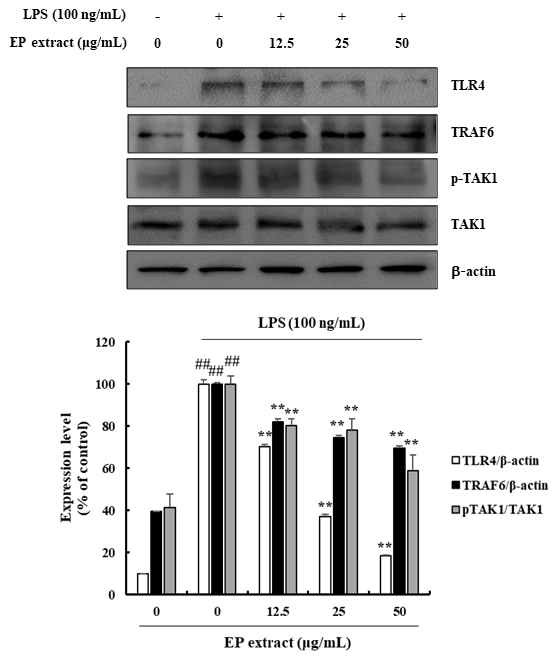
Effects of EP extract on TLR4 in LPS-stimulated RAW264.7 cells. RAW264.7 cells were stimulated with LPS (100 ng/mL) in the absence or presence of EP extract (12.5, 25, or 50 μg/mL) for 24 h. Protein expressions were analyzed by western blot with specific antibodies. β-actin or TAK1 were used as controls. The data presented are the mean ± standard deviation of three independent experiments and differences between the mean values. ##P< 0.01 vs. Control group; *P< 0.05, **P< 0.01 vs. LPS group.
Discussion
The innate immune system triggers a rapid inflammatory response in the early stages of infection, repressing the growth and spread of the infectious pathogens.2,20) LPS, a major composition of the outer membrane of Gram-negative bacteria potently activates macrophages through TLR4 and can induce various pro-inflammatory mediators and cytokines through distinct signaling pathways.10) These inflammatory responses underlie various physiological and pathological processes.21) Based on this knowledge, novel anti-inflammatory agents based on the suppression of pro-inflammatory cytokines and mediator generation, and related signal transduction have been tested.10)
In this study, we explored the anti-inflammatory effects and elucidated the potential molecule mechanism of EP extract. First, we examined the possibility of EP extract to inhibit NO production. Our results revealed that EP extract treatment decreased the LPS-mediated NO production. Many studies have elucidated that the macrophages secrete pro-inflammatory cytokines in the early stages of pathogenic stimulated inflammation and that excessive production of pro-inflammatory cytokines expand the range of immune responses.22-24) Based on this, we further evaluated the inhibitory effect of EP extract on pro-inflammatory cytokine expression in LPS-treated RAW264.7 cells. Our results showed that EP extract significantly suppressed the production of iNOS, IL-2, IL-6, IL-1β, IFN-γ, and TNF-α without any cytotoxicity in RAW264.7 cells. We also confirmed the presence of anti-inflammatory cytokines such as IL-10 in LPS-stimulated RAW264.7 cells. IL-10 was significantly increased in EP extract-treated RAW264.7 cells when compared with only LPS-stimulated cells. These results clearly demonstrated that the EP extract anti-inflammatory activity in LPS-induced inflammation in RAW264.7 cells.
NF-κB and MAPKs are the most crucial signaling pathways in LPS-induced inflammatory responses. LPS binding to TLR4 on the macrophage membrane initiates LPS-mediated NF-κB signaling.25) TLR4 bound to LPS induces activation and translocation of NF-κB to the cell nucleus, where it binds to its DNA binding site, promoting the transcription of inflammatory genes including the iNOS, IL-2, IL-6, IL-1β, IFN-γ, and TNF-α.26,27) The present study found that EP extract suppressed LPS-induced phosphorylation of IKKαβ, IκB, and NF-κB p65 indicating that EP extract suppresses LPS-mediated inflammation by inhibiting the NF-κB signaling pathway.
In the mammalian species, the MAPK family includes ERK, JNK, and p38 sub-families (MAPKs). The LPS-induced inflammation activates the phosphorylation of these MAPKs in RAW264.7 cells, which also upregulates the expression of inflammatory cytokines.28-34) In the present study, EP extract prominently inhibited LPS-induced phosphorylation of ERK and p38 MAPK in RAW264.7 cells. These results reveal that the anti-inflammatory effects of EP extract are mediated by the blockade of ERK and p38 MAPK signaling, along with suppression of NF-κB signaling, in LPS-treated RAW264.7 cells.
TLR4 is a family of proteins that plays an essential role in the innate immune system, in which TLR-mediated signaling pathway activates the NF-κB and MAPK pathways resulting in the production of pro-inflammatory cytokines.35-40) TLR4 signaling leads to downstream activation of TRAF6, to form a complex composed of TAK1 and TAB proteins, which activates TAK1 by autophosphorylation. TAK1 is a crucial upstream factor of the NF-κB and MAPK signaling.41-43) In the present study, we investigated whether EP extract suppresses LPS-mediated inflammation through TLR4 signaling. Based on our findings, EP extract decreased LPS-mediated expression of TLR4, TRAF6 and phosphorylation of TAK1 in RAW264.7 cells.
In the present study, we confirmed the EP extract anti-inflammatory effects by demonstrating that EP extract inhibited the expression of pro-inflammatory cytokines in LPS-induced RAW264.7 cells. Furthermore, the inhibitory effect of EP extract involved suppression of TLR4-mediated NF-κB and MAPKs signaling pathways. Taken together, our results demonstrated that EP extract as a potential anti-inflammatory agent. In addition, our results suggested that the EP extract exert the anti-inflammatory effects through inhibition of the TLR4 signaling pathway.
Acknowledgments
The authors thank Evear Extraction, Féline, France for providing samples, encouragement, and generous support.
References
-
Medzhitov R, Janeway JrC. Innate immunity. N Engl J Med. 2000;343(5):338-44.
[https://doi.org/10.1056/NEJM200008033430506]
-
Park BS, Lee JO. Recognition of lipopolysaccharide pattern by TLR4 complexes. Exp Mol Med. 2013;45(12):e66.
[https://doi.org/10.1038/emm.2013.97]
-
Janeway JrCA, Medzhitov R. Innate immune recognition. Annu Rev Immunol. 2002;20(1):197-216.
[https://doi.org/10.1146/annurev.immunol.20.083001.084359]
-
Medzhitov R, Janeway JrC. Innate immune recognition: mechanisms and pathways. Immunol Rev. 2000;173:89-97.
[https://doi.org/10.1034/j.1600-065X.2000.917309.x]
-
Janeway CA. Approaching the asymptote? Evolution and revolution in immunology. Cold Spring Harb Symp Quant Biol. 1989;54:1-13.
[https://doi.org/10.1101/SQB.1989.054.01.003]
-
Scott MG, Gold MR, Hancock RE. Interaction of cationic peptides with lipoteichoic acid and gram-positive bacteria. Infect Immun. 1999;67(12):6445-53.
[https://doi.org/10.1128/IAI.67.12.6445-6453.1999]
-
Cheon SY, Chung KS, Jeon E, Nugroho A, Park HJ, An HJ. Anti-inflammatory activity of saxifragin via inhibition of NF-κB involves caspase-1 activation. J Nat Prod. 2015;78(7):1579-85.
[https://doi.org/10.1021/acs.jnatprod.5b00145]
-
Maruthamuthu V, Henry LJK, Ramar MK, Kandasamy R. Myxopyrum serratulum ameliorates airway inflammation in LPS-stimulated RAW 264.7 macrophages and OVA-induced murine model of allergic asthma. J Ethnopharmacol 2020;255:112369.
[https://doi.org/10.1016/j.jep.2019.112369]
-
Barton GM. A calculated response: control of inflammation by the innate immune system. Journal Clin Investg. 2008;118(2):413-20.
[https://doi.org/10.1172/JCI34431]
-
Lu YC, Yeh WC, Ohashi PS. LPS/TLR4 signal transduction pathway. Cytokine 2008;42(2):145-51.
[https://doi.org/10.1016/j.cyto.2008.01.006]
-
Beutler B. Tlr4: central component of the sole mammalian LPS sensor. Curr Opin Immunol. 2000;12(1):20-6.
[https://doi.org/10.1016/S0952-7915(99)00046-1]
-
Ghosh S, May MJ, Kopp EB. NF-κB and Rel proteins: evolutionarily conserved mediators of immune responses. Annu Rev Immunol. 1998;16(1):225-60.
[https://doi.org/10.1146/annurev.immunol.16.1.225]
-
Verma IM, Stevenson JK, Schwarz EM, Van Antwerp D, Miyamoto S. Rel/NF-kappa B/I kappa B family: intimate tales of association and dissociation. Genes Dev. 1995;9(22):2723-35.
[https://doi.org/10.1101/gad.9.22.2723]
- Rao KMK. MAP kinase activation in macrophages. J Leukoc Biol. 2001;69(1):3-10.
-
Kim EK, Choi EJ. Pathological roles of MAPK signaling pathways in human diseases. Biochim Biophys Acta Mol Basis Dis. 2010;1802(4):396-405.
[https://doi.org/10.1016/j.bbadis.2009.12.009]
-
Craig WJ. Health-promoting properties of common herbs. Am J Clin Nutr. 1999;70(3):491s-99s.
[https://doi.org/10.1093/ajcn/70.3.491s]
- Kumar KM, Ramaiah S. Pharmacological importance of Echinacea purpurea. Int J Pharma Bio Sci. 2011;2(4):304-14.
-
Woelkart K, Linde K, Bauer R. Echinacea for preventing and treating the common cold. Planta Med. 2008;74(06):633-7.
[https://doi.org/10.1055/s-2007-993766]
-
Yamada K, Hung P, Park TK, Park PJ, Lim BO. A comparison of the immunostimulatory effects of the medicinal herbs Echinacea, Ashwagandha and Brahmi. J Ethnopharmaco. 2011;137(1):231-5.
[https://doi.org/10.1016/j.jep.2011.05.017]
-
Axen A, Carlsson A, Engström Å, Bennich H. Gloverin, an antibacterial protein from the immune hemolymph of Hyalophora pupae. Eur J Biochem. 1997;247(2):614-619.
[https://doi.org/10.1111/j.1432-1033.1997.00614.x]
-
Medzhitov R. Origin and physiological roles of inflammation. Nature. 2008;454(7203):428-35.
[https://doi.org/10.1038/nature07201]
-
Zhao G, Wu H, Jiang K, Chen X, Wang X, Qiu C, et al. The anti-inflammatory effects of interferon tau by suppressing NF-κB/MMP9 in macrophages stimulated with Staphylococcus aureus. J Interferon Cytokine Res. 2016;36(8):516-24.
[https://doi.org/10.1089/jir.2015.0170]
-
Wang T, Hou W, Fu Z. Preventative effect of OMZ-SPT on lipopolysaccharide-induced acute lung injury and inflammation via nuclear factor-kappa B signaling in mice. Biochem Biophys Res Commun. 2017;485(2):284-9.
[https://doi.org/10.1016/j.bbrc.2017.02.090]
-
Park JR, Lee H, Kim SI, Yang SR. The tri-peptide GHK-Cu complex ameliorates lipopolysaccharide-induced acute lung injury in mice. Oncotarget. 2016;7(36):58405.
[https://doi.org/10.18632/oncotarget.11168]
-
Olefsky JM, Glass CK. Macrophages, inflammation, and insulin resistance. Annu Rev Physiol. 2010;72:219-46.
[https://doi.org/10.1146/annurev-physiol-021909-135846]
-
Surh YJ, Chun KS, Cha HH, Han SS, Keum YS, Park KK, et al. Molecular mechanisms underlying chemopreventive activities of anti-inflammatory phytochemicals: down-regulation of COX-2 and iNOS through suppression of NF-κB activation. Mutat Res-Fundam Mol Mech Mutagen. 2001;480:243-68.
[https://doi.org/10.1016/S0027-5107(01)00183-X]
-
Gil A, Aguilera CM, Gil-Campos M, Canete R. Altered signalling and gene expression associated with the immune system and the inflammatory response in obesity. Br J Nutr. 2007;98(S1):S121-6.
[https://doi.org/10.1017/S0007114507838050]
-
Zhimin L, Tony H. Degradation of activated protein kinases by ubiquitination. Annu Rev Biochem. 2009;78:435-75.
[https://doi.org/10.1146/annurev.biochem.013008.092711]
-
Weinstein SL, Sanghera JS, Lemke K, DeFranco AL, Pelech SL. Bacterial lipopolysaccharide induces tyrosine phosphorylation and activation of mitogen-activated protein kinases in macrophages. J Biol Chem. 1992;267(21):14955-62.
[https://doi.org/10.1016/S0021-9258(18)42133-3]
- Sanghera JS, Weinstein SL, Aluwalia M, Girn J, Pelech SL. Activation of multiple proline-directed kinases by bacterial lipopolysaccharide in murine macrophages. J Immunol. 1996;156(11):4457-65.
-
Swantek JL, Cobb MH, Geppert TD. Jun N-terminal kinase/stress-activated protein kinase (JNK/SAPK) is required for lipopolysaccharide stimulation of tumor necrosis factor alpha (TNF-alpha) translation: glucocorticoids inhibit TNF-alpha translation by blocking JNK/SAPK. Mol Cell Biol. 1997;17(11):6274-82.
[https://doi.org/10.1128/MCB.17.11.6274]
- Chen CC, Wang JK. p38 but not p44/42 mitogen-activated protein kinase is required for nitric oxide synthase induction mediated by lipopolysaccharide in RAW 264.7 macrophages. Mol Pharmacol. 1999;55(3):481-8.
-
Arthur JSC, Ley SC. Mitogen-activated protein kinases in innate immunity. Nat Rev Immunol. 2013;13(9):679-92.
[https://doi.org/10.1038/nri3495]
-
Roget K, Ben-Addi A, Mambole-Dema A, Gantke T, Yang HT, Janzen J, et al. IκB kinase 2 regulates TPL-2 activation of extracellular signal-regulated kinases 1 and 2 by direct phosphorylation of TPL-2 serine 400. Mol Cell Biol. 2012;32(22):4684-90.
[https://doi.org/10.1128/MCB.01065-12]
-
Lu YC, Yeh WC, Ohashi PS. LPS/TLR4 signal transduction pathway. Cytokine. 2008;42(2):145-51.
[https://doi.org/10.1016/j.cyto.2008.01.006]
-
Poltorak A, He X, Smirnova I, Liu MY, Van Huffel C, Du X, et al. Defective LPS signaling in C3H/HeJ and C57BL/10ScCr mice: mutations in Tlr4 gene. Science. 1998;282(5396):2085-8.
[https://doi.org/10.1126/science.282.5396.2085]
-
Qian Y, Commane M, Ninomiya-Tsuji J, Matsumoto K, Li X. IRAK-mediated translocation of TRAF6 and TAB2 in the interleukin-1-induced activation of NFκB. J Biol Chem. 2001;276(45):41661-7.
[https://doi.org/10.1074/jbc.M102262200]
-
Baud V, Liu ZG, Bennett B, Suzuki N, Xia Y, Karin M. Signaling by proinflammatory cytokines: oligomerization of TRAF2 and TRAF6 is sufficient for JNK and IKK activation and target gene induction via an amino-terminal effector domain. Genes Dev. 1999;13(10):1297-1308.
[https://doi.org/10.1101/gad.13.10.1297]
-
Cao Z, Xiong J, Takeuchi M, Kurama T, Goeddel DV. TRAF6 is a signal transducer for interleukin-1. Nature. 1996;383(6599):443-6.
[https://doi.org/10.1038/383443a0]
-
Lomaga MA, Yeh WC, Sarosi I, Duncan GS, Furlonger C, Ho A, et al. TRAF6 deficiency results in osteopetrosis and defective interleukin-1, CD40, and LPS signaling. Genes Dev. 1999;13(8):1015-24.
[https://doi.org/10.1101/gad.13.8.1015]
-
Jadhav T, Geetha T, Jiang J, Wooten MW. Identification of a consensus site for TRAF6/p62 polyubiquitination. Biochem Biophys Res Commun. 2008;371(3):521-4.
[https://doi.org/10.1016/j.bbrc.2008.04.138]
-
Yang K, Zhu J, Sun S, Tang Y, Zhang B, Diao L, et al. The coiled-coil domain of TRAF6 is essential for its auto-ubiquitination. Biochem Biophys Res Commun. 2004;324(1):432-9.
[https://doi.org/10.1016/j.bbrc.2004.09.070]
-
Chiu YH, Zhao M, Chen ZJ. Ubiquitin in NF-κB Signaling. Chem Rev. 2009;109(4):1549-60.
[https://doi.org/10.1021/cr800554j]