
황금탕의 족돌기세포에서의 EMT 억제 효능
Ⓒ The Society of Pathology in Korean Medicine, The Physiological Society of Korean Medicine
Abstract
Epithelial-mesenchymal transition (EMT) is the process by which epithelial cells lose their characters and acquire the properties of mesenchymal cells. EMT has been reported to exert an essential role in embryonic development. Recently, EMT has emerged as a pivotal mechanism in the metastasis of cancer and the fibrosis of chronic diseases. In particular, EMT is drawing attention as a mechanism of renal fibrosis in chronic kidney diseases such as diabetic nephropathy. In this study, we developed an EMT model by treating TGF-β1 on the podocytes, which play a key role in the renal glomerular filtration. This study explored the effects of Hwanggeum-tang (HGT) recorded in Dongeuibogam as being able to be used for the treatment of Sogal whose concept had been applied to Diabetes Mellitus (DM), on the TGF-β1-induced podocyte EMT. HGT suppressed the expression of vimentin and α-SMA, the EMT marker, in the human podocytes stimulated by TGF-β1. However, HGT increased the expression of ZO-1 and nephrin. Interestingly, HGT selectively inhibited the mTOR pathway rather than the classical Smad pathway. HGT also activated the AMPK signaling. HGT’s inhibitory effect on the podocyte EMT through regulation of the mTOR pathway was achieved through the activation of AMPK, which was confirmed by comparison with cells treated with compound C (CC), an inhibitor of AMPK signaling. In conclusion, HGT can be applied to the renal fibrosis by preventing TGF-β1-induced EMT of podocytes through AMPK activation and mTOR inhibition.
Keywords:
Epithelial–mesenchymal transition, Kidney fibrosis, mTOR, AMPK서 론
신장의 섬유화는 대부분의 만성 신장질환에서 공통적으로 관찰할 수 있는 현상이다1). 신장섬유화의 기본 메커니즘을 밝히고자 많은 연구들이 진행되었으나, 신장 손상에 따른 섬유화 기전은 아직 완전히 밝혀져 있지 않았으며, 만성 신장 섬유화를 효과적으로 억제하거나 치료 전략을 수립하고자 함에 있어 섬유화 기전에 대한 연구는 필수적이다.
최근에는 당뇨병성 신장질환을 비롯한 만성 신장질환에서 신장의 섬유화에 대한 기전 가운데 상피중간엽 전이 (epithelial mesenchymal transition; EMT)가 주목을 받고 있다. 완전히 분화된 상피세포가 섬유아세포의 표현형으로 전이되는 과정인 EMT는 신장 손상에 따른 섬유아세포의 생성에 중요한 경로로 대두되고 있다2,3).
본래 EMT 배아의 발달과정과 종양의 전이과정에서 핵심적 역할을 하는 경로로 주목받아 왔으나 최근 연구에서는 신장 섬유화를 초래하는 만성 병변에서 EMT의 관찰이 보고되었고, 현재 EMT는 신장 섬유화 기전에서 가장 흥미로운 주제 중 하나로 주목받고 있다4).
신장의 핵심기능인 여과를 담당하고 있는 사구체는 여러 종류의 세포들로 이루어져 있다. 그 가운데 족돌기세포 (podocyte)는 사구체 여과에서 핵심적인 역할을 담당한다5). 족돌기세포의 손상은 단백뇨의 발생을 유도하고, 사구체경화증에 이어서 신장기능의 소실까지 유도할 수 있다6). 따라서 족돌기세포의 손상 기전을 연구하는 것은 신장기능소실로의 진행을 예방하거나 늦추는 전략을 개발하는데 있어서 매우 중요한 과정이다.
만병회춘과 동의보감 소갈문에 기재되어 있는 황금탕은 당뇨병으로 인한 혈관 합병증에 응용될 수 있는 것으로 보고되었다7). 본 연구에서는 족돌기세포에 TGF beta로 EMT를 유도하였고, 소갈문의 처방인 황금탕이 이를 억제하는 효능이 있는지 여부를 확인한 결과 유의미한 결과를 도출하였기에 보고하는 바이다.
재료 및 방법
1. 세포배양
인간 족돌기세포는 Bristol university로부터 분양받았다. 족돌기세포는 RPMI 1640 medium에 10% fetal bovine serum (FBS), 1% penicillin/streptomycin, 1% insulin을 첨가하여 33 ℃ incubator에서 maintain하였으며, 37 ℃ incubator로 옮긴 후 2% FBS, 1% penicillin/streptomycin을 첨가한 RPMI 1640 medium에서 2주간 분화시킨 후 사용하였다.
2. HGT 추출
본 연구에서 이용한 HGT 구성은 동의보감 소갈문에 준하였으며, 본 연구팀에서 기존에 발표했고, 현재 보유하고 있는 HGT추출물을 이용하였다. 추출방법은 기존 연구에서 상세히 기술하였다7). HGT의 한 첩당 구성약재비율은 다음과 같다. Scutellariae Radix(황금), Gardeniae Fructus(치자), Platycodi Radix(길경), Liriopis Tuber (맥문동), Angelicae Gigantis Radix (당귀), Rehmannia glutinosa (생지황), Trichosanthis Radix (천화분), Puerariae Radix (갈근), Ginseng Radix (인삼), Paeoniae Radix Alba (백작약) 각 4g, 총 40g.
3. 세포독성반응 측정
HGT의 세포독성 반응을 측정하기 위하여 살아있는 세포의 미토콘드리아 전자전달계 내 dehydrogenase와 반응하여 수용성 formazan을 생성하는 tetrazolium salt인 EZ-cytox (㈜대일, 대한민국)를 이용하였다. 측정법을 간략히 서술하면 다음과 같다. Podocytes를 12 well plate에 well 당 1X 105개로 분주하여 37 ℃ incubator 에서 2주를 분화시킨 후 HGT추출물을 농도별로 24시간 처리하였다. 이후 EZ-Cytox 100 ㎕를 각 well에 첨가한 후 30분간 incubator에서 반응시켰다. 이 후 microplate reader를 이용하여 450 ㎚에서 흡광도를 측정하였다.
4. Western blot
Human podocytes를 6 well plate에 ml 당 1X 105개로 분주(2X 105 개/well)하여 37 ℃ incubator 에서 2주를 분화시킨 후 실험을 진행하였다. 단백질 추출은 mild lysis buffer (10 mM Tris, pH 7.5, 2 mM EDTA, 100 mM NaCl, 1% Triton X-100, 50 mM NaF)를 이용하였다. 6 well plate를 얼음 위에 놓고, mild lysis buffer 120 ㎕를 넣고 4 ℃ cold room에서 1500 rpm microplate shaker 위에서 10분간 세포를 lysis 시켰다. Cell lysates를 1.5 ml tube 에 모집한 후 4 ℃ 에서 14,000 rpm 으로 15분간 원심분리하여 상층액을 수집하였다. 이 후 Bradford 방법으로 단백질을 정량한 후 western blot을 시행하였다. SDS-page gel에서 전기영동을 한 후 분리된 단백질은 PVDF membrane으로 90분동안 transfer하였다. 이 후 5% non-fat dry milk에서 1시간 동안 blocking시킨 후 1차 항체를 4 ℃에서 overnight으로 반응시켰다. 다음날 TBST로 10분간 3회 세척한 후 HRP가 결합된 2차 항체와 상온에서 1시간 반응켰고, 이 후 TBST로 10분간 4회 세척 후 ECL solution을 이용하여 발색하고, RAS기기를 통하여 밴드를 확인하였다. 실험에 사용된 항체의 정보는 다음과 같다. Nephrin, α-SMA (Abcam, Cambridge, UK), pACC, ACC, pAkt, Akt, pmTOR, mTOR, pS6K, S6K, pSmad2, Smad2/3, pJNK, JNK, pp38, p38, vimentin (Cell Signaling Technology, Inc, MA, USA), GAPDH, β-actin (Santa Cruz Biotechnology Inc. TX, USA) ZO-1 (Invitrogen Corporation, CA, USA).
5. Confocal microscopy
12 well plate에 cover glass를 덮고 human podocyte를 well 당 1X 105개로 분주하여 33 ℃ incubator에서 하루를 배양하였고, 37 ℃ incubator로 옮겨 2주간 분화시킨 후 사용하였다. HGT를 농도별로 16시간 처리하고, TGF β-1 (10 ng/ml) 를 24시간 처리한 후 세포를 PBS로 세척하였다. 이 후 4% formaldehyde solution으로 10분 간 고정하였고, PBS로 세척한 후 0.1% Triton X-100/PBS solution 세포막의 투과성을 확보하였다. 이 후 3% BSA/PBS solution으로 30분 동안 blocking한 후 세포를 ZO-1 antibody (1:200 dilution)로 4℃에서 overnight하여 반응시켰다. 다음날 PBS로 10분간 3회 세척한 후 anti-Rabbit Alexa Fluor 488 antibody로 1시간 반응시킨 후 PBS로 세척하고, 이어서 ProLong® Gold Antifade Mountant with DAPI로 mounting하였다. 다음날 아침 nail polish 로 sealing하여 slide glass를 굳힌 후 Zeiss LSM 700 Laser Scanning Confocal microscopy로 이미지를 얻었다.
결 과
1. 세포생존율
HGT가 족돌기세포에 독성을 가지고 있는지 우선 살펴보고자 EZ-cytox kit를 이용하여 세포생존율을 관찰하였다. 분화가 완료된 족돌기세포에 HGT를 농도별로 24 시간 처리하고 세포독성을 조사하였다. HGT는 100 μg/ml의 농도까지는 세포독성을 보이지 않았다. 이를 반영하여 세포 독성이 없는 농도에서 이 후 실험을 진행하였다.
2. HGT가 족돌기세포에서 EMT 마커의 발현에 미치는 영향
당뇨병성 신병증에서 족돌기세포의 특징적 변화 중 하나인 EMT에 HGT가 미치는 영향을 관찰하고자, 분화된 족돌기세포에 HGT를 농도별로 16시간 동안 선처리 한 후 TGF-β1 (10 ng/ml)을 24시간 처리하여 EMT를 유도하였다. 이후 western bot과 confocal microscope를 이용하여 여러 가지 EMT 마커들의 발현변화를 관찰하였다. TGF-β1의 처리는 족돌기세포에서 EMT 마커인 vimentin과 αSMA의 발현을 증가시킨 반면, 상피세포 마커이자 족돌기세포의 여과기능에서 핵심적인 역할을 하는 것으로 잘 알려진 nephrin과 상피세포 마커인 ZO-1의 발현은 감소시켰다. HGT의 투여는 TGF-β1에 의해 증가된 vimentin과 αSMA의 발현을 억제하였으며, TGF-β1에 의해 감소된 nephrin과 zonula occludens-1(ZO-1)의 발현은 유지시켰다 (Fig. 2A). Nephrin과 더불어 상호작용을 하면서 족돌기세포의 기능조절에 핵심적인 역할을 담당하는 것으로 알려진 ZO-1의 세포막에서의 발현은 TGF-β1의 처리에 의해 현저히 감소되었고, EMT marker인 vimentin의 세포질에서의 발현은 증가하였다. HGT의 선처리는 TGF-β1에 의한 이들의 발현 변화를 모두 억제하였다 (Fig. 2B).
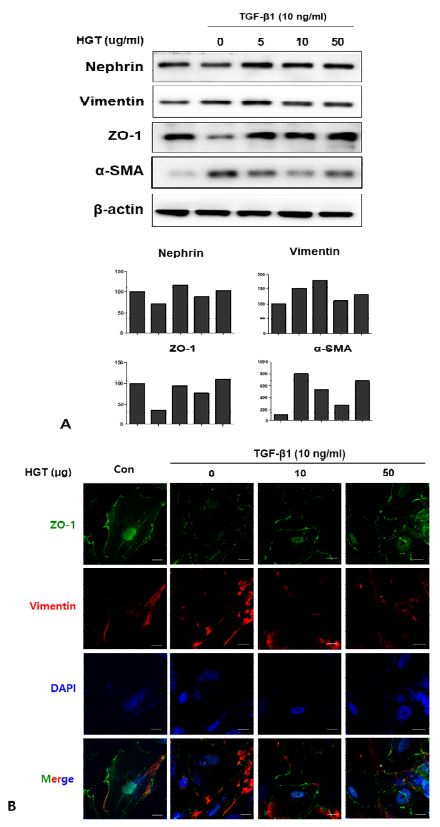
HGT inhibited EMT in TGF-β1-treated podocytes. A. TGF-β1 increased EMT marker while decreased epithelial marker in human podocytes. HGT treatment suppressed the expression of vimentin and α-SMA, the EMT marker in human podocytes stimulated by TGF-β1. HGT also increased the expression of ZO-1 and nephrin, the key molecule in the function of podocytes. B. The expression of ZO-1 on the cell membrane was markedly decreased by TGF-β1 in human podocytes, while it was prevented by pretreatment of HGT. The expression of vimentin in the cytoplasm was increased by TGF-β1; however, the increased expression of vimentin by TGF-β1 was suppressed by HGT.
3. HGT가 podocyte에서 EMT관련 신호전달경로에 미치는 영향
TGF-β1이 EMT를 유도하는 대표적인 신호전달경로는 전형적인 Smad 경로이다. 이 외에도 TGF- β1의 신호전달경로로 PI3kà Aktà mTOR 경로와 JNK, p38과 같은 MAPK경로가 알려져 있다. 이에 연구진은 HGT의 TGF-β1로 유도한 족돌기세포의 EMT억제 효능이 어떤 경로를 통하는지 살펴보고자 위의 세 신호전달 경로를 western blot을 통하여 확인해 보았다. 매우 흥미롭게도 HGT는 전형적인 Smad경로와(Fig. 3B) JNK, p38경로는 억제하지 않은 반면(Fig. 3C), mTOR경로를 선택적으로 억제하였다(Fig. 3A).
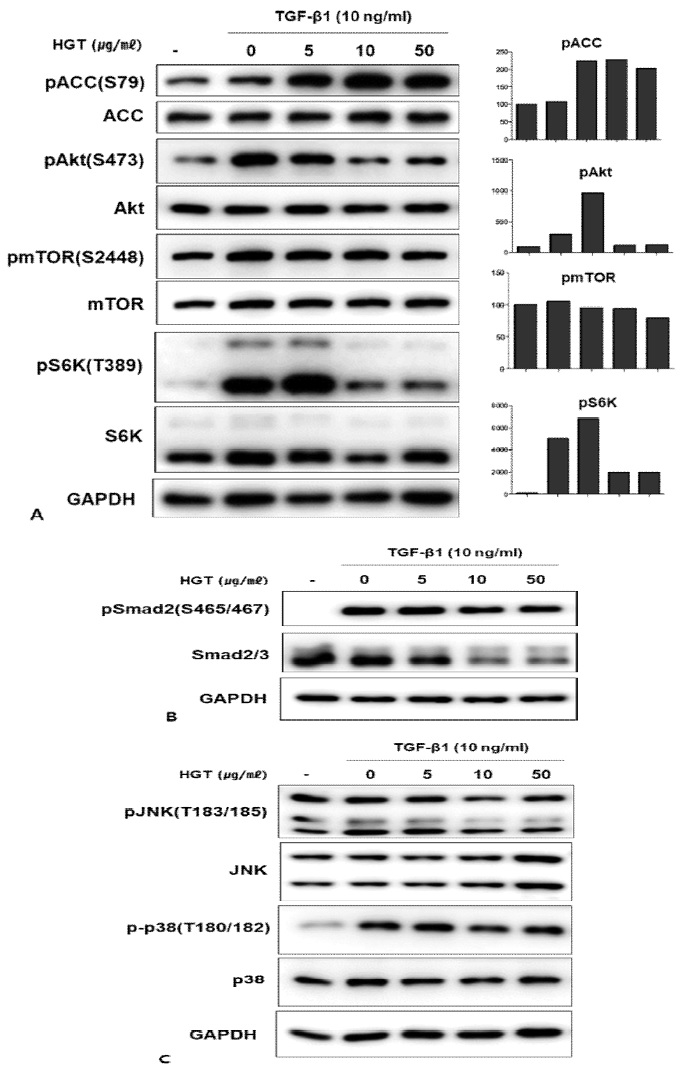
HGT inhibited mTOR signaling in TGF-β1-treated podocytes. A. TGF-β1 activated mTOR signaling. HGT inhibited mTOR signaling as confirmed by the decreased expression of p-Akt, p-mTOR, p-S6K, especially in high doses (10, 50 μg/ml). Interestingly, HGT activated the AMPK pathway, confirmed by the increased expression of p-ACC. However, HGT had little effect on the smad and MAPK pathways (B, C).
4. HGT의 AMPK활성에 의한 EMT억제 기전
HGT는 mTOR의 상위에서 억제자인 TSC2을 활성화시키고, mTORC1의 raptor를 인산화시킴으로써 직접적으로 mTOR의 활성을 억제하는 것으로 잘 알려진 AMPK의 신호전달 경로를 강력히 활성화시키는 것을 AMPK의 대표적인substrate인 ACC의 인산화를 통하여 확인하였다 (Fig. 3A). 이에 본 연구진은 HGT에 의한 AMPK의 활성화가 mTOR억제를 통한 EMT억제에 어느 정도의 기여를 하는지 관찰하고자, AMPK의 대표적인 억제제인 compound C를 처리한 세포에서 EMT의 변화를 살펴보았다. 흥미롭게 vimentin과 α-SMA의 발현 억제효능은 compound C를 처리한 군에서 두드러지게 감소하였다 (Fig. 4A, B). 이는 HGT가 podocyte 에서 EMT 마커인 vimentin과 α-SMA의 발현을 억제하는 능력이 HGT의 AMPK활성에 의하여 이루어지고 있음을 시사한다.
고 찰
제 1형 및 2형 전체 당뇨병 환자의 25-40%에서 합병증으로 발생하는 것으로 알려진 당뇨병성 신병증 (Diabetic nephropathy, DN)은8) 투석이나 신장이식을 요구하는 만성신부전의 가장 큰 원인질환으로 주목받고 있다9). DN의 가장 특징적인 병리변화는 사구체 메산지움이나 사이질에 세포외기질 (extracellular matrix, ECM)이 축적되어 신장의 점진적인 기능소실과 신장의 섬유화를 유도하는 것이다8,10). 신장 세관과 사이질의 섬유화 정도에 비례하여 신장의 기능부전이 발생하는 것으로 보고되고 있다11).
보우만주머니 내의 장측상피세포인 족돌기세포는 사구체기저막의 바깥부분에 부착되어 있다. 족돌기세포는 laminin, type IV collagen, fibronectin, agrin, heparin sulphate proteoglycan 등의 사구체기저막 성분들을 합성함으로써 기저막의 구조를 유지하는 역할을 한다12). 이 세포에는 발가락모양과 유사하다고 하여 족돌기(foot process)라고 부르는 수많은 세포질 돌기들이 존재한다. 족돌기세포의 족돌기들은 인접한 족돌기세포의 족돌기들과 연계되어 틈새가로막 (slit diaphragm)이라는 구조물을 형성하고 있으며, 이는 신장의 여과기능에 핵심적인 역할을 담당한다13,14).
DN이 진행되고 있음을 시사하는 요단백의 증가는 족돌기세포의 비대, EMT, 기저막으로부터의 탈착, 세포자멸사 등의 병리변화와 연관되어 있다15). 많은 선행연구에서 AMPK, mTOR, ER-stress 등의 다양한 신호전달경로의 혼란이 이러한 DN의 진행에 핵심적인 과정으로 보고되고 있다16). 특히, 만성 신장질환에서의 상피중간엽전이 (Epithelial‐mesenchymal transition, EMT)의 역할은 Iwano의 연구17) 이후 널리 보고되고 있다. 신장질환을 앓고 있는 환자의 신장조직을 이용한 연구에서 근섬유모세포들이 증가하고, EMT과정을 거치는 세포들의 비율이 신장의 섬유화 정도와 혈청 크레아티닌 정도에 비례하여 증가된다고 보고되었다18,19). 또한 신장질환을 유도한 마우스모델에서도 당화종말산물이나 profibrotic cytokine, 성장인자 등을 처리할 경우 EMT가 발생한다고 보고되었다20).
TGF-β는 많은 신장세포에서 분비되며, Smad, MAPK 등 다양한 신호전달과정을 거치는 것으로 알려져 있다21). 사구체 세포의 염증성 사이토카인에 대한 반응을 최소화하거나 사구체로의 염증세포의 유입을 막는 등 유익한 작용도 가지고 있으나, DN을 포함한 다양한 사구체질환에서 TGF-β는 사구체경화증을 발생시키는데 핵심적인 역할을 하는 것으로 잘 알려져 있다22). 만성신장질환에서 TGF-β의 분비는 증가되어 있으며, 이들은 신장세포에 작용하여 세포외기질을 생산하도록 유도함으로써 사구체경화증과 신장 섬유화를 일으키며, 족돌기세포의 족돌기소실 및 세포자멸사를 일으킴으로써 신장기능을 감소시키는데 중요한 역할을 하는 것으로 밝혀졌다23). 세 가지 isoform(TGF-β1, -β2, -β3) 가운데 TGF-β1은 당뇨합병증에서 ECM의 침착을 유도하는 핵심적인 매개체로 알려져 있다24).
TGF-β는 세포 표면 수용체를 통하여 기능을 수행하는데 type I 수용체와 type II 수용체는 모두 transmembrane serine/threonine kinase들이다. 대부분의 세포에서 TGF-β 는 먼저 type II 수용체에 결합하며, 이들의 결합체를 type I 수용체가 인식하여 이들 결합체에 binding을 하게 된다. Type I수용체는 type II수용체에 의하여 인산화가 된 후, 전형적인 Smad 신호전달계를 거친다25). TGF-β는 전형적인 Smad pathway이외에 phosphatidylinositol 3-kinase, Akt를 통한 mammalian target of rapamycin (mTOR)을 활성화시킴으로써 EMT를 유도하는 것으로 보고되었다26). mTOR는 세린/크레오닌 키나아제로 세포의 크기와 부피, 증식, 생존에 있어서 중추적인 역할을 하는 것으로 알려져 있다27). 세포에는 mTOR complex1(mTORC1)과 mTOR complex2(mTORC2)가 존재하며, 이 중 mTORC1은 전사 이후의 번역(translation)과정의 조절함으로써 세포의 크기와 부피를 증식시키는 것으로 잘 알려져 있다. 많은 종양과 대사상질병에서 mTOR의 활성이 증가되어 있으며, 이를 특이적으로 억제하는 rapamycin과 같은 약물은 이들의 치료에 응용될 수 있다28). 당뇨병성 신병증에서 나타나는 구조적 변화 중 하나인 신장의 증식은 사구체와 세관상피세포의 비대로 인한다. 기존에 보고된 많은 연구들에 의하면 당뇨병성 신병증의 신장 비대에 mTOR의 활성화가 중추적 역할을 하는 것으로 밝혀졌다29,30).
본 연구에서는 TGF-β1으로 EMT를 유도한 족돌기세포를 이용하여 실험을 진행하였다. HGT는 족돌기세포에서 TGF-β1처리로 인한 상피세포마커인 ZO-1과 nephrin의 발현 감소를 억제한 반면, 중간엽 마커인 vimentin과 α-SMA의 증가는 감소시켰다(Fig. 2). 이로써 HGT가 족돌기세포에서의 TGF-β1로 유도한 EMT를 억제하는 효과가 있음을 확인하였다. 이어서 본 연구진은 HGT의 EMT 억제 효능의 구체적인 기전을 탐색하고자, TGF-β1의 신호전달경로를 탐색하였다. 흥미롭게도 HGT는 전형적인 Smad경로나 MAPK 경로를 억제하지 않은 반면(Fig. 3B, C), AktàmTOR 경로를 선택적으로 억제하였고, AMPK경로를 활성화시켰다(Fig. 3A). 에너지 스트레스에 노출되어 세포 내 ATP가 떨어지고 AMP가 높아지는 상황에서 활성화되어 catabolism을 유도하는 AMPK는 mTOR상위에 존재하는 TSC2를 활성화시키고, 아울러 mTORC1의 raptor를 인산화시킴으로써 mTOR 신호전달을 억제하는 것으로 잘 알려져 있다31). 이에 연구진은 HGT의 AMPK를 활성화시키는 능력이 mTOR억제를 통한 EMT방어 효능에 어느 정도의 역할을 담당하고 있는지 확인하고자, AMPK inhibitor인 compound C (CC)를 처리한 세포와 비교 관찰을 하였다. 실험결과 CC를 처리한 군에서 HGT는 vimentin와 α-SMA의 발현을 억제시키지 못하였다. 이 결과는 HGT의 EMT억제능력이 AMPK의 활성에 의존하고 있음을 시사한다.
이상의 실험결과를 종합해 보면, 동의보감 소갈문의 HGT는 TGF-β1을 처리한 신장의 족돌기세포에서 TGF-β1의 여러 신호전달 경로 중 mTOR 신호경로를 선택적으로 억제하였으며, AMPK 또한 활성화시킴으로써 EMT를 억제하였다. 따라서 동의보감 소갈문에 기재된 HGT는 신장부전을 유도하는 가장 대표적 질환인 DN에서의 신장의 섬유화를 억제하는데 응용할 수 있을 것으로 기대한다.
Acknowledgments
본 연구는 부산대학교 기본연구지원사업 (2년)의 지원을 받아 수행되었음.
References
-
Abstracts of the 7th Lung Cancer Biology Workshop: Molecular biology and pharmacogenetic research in the treatment of lung cancer, Barcelona, Spain, April 30 May 3, 2001. Lung Cancer. 2001;34(3):S1-S26.
[https://doi.org/10.1016/S0169-5002(01)00418-4]
-
Liu YH. New Insights into Epithelial-Mesenchymal Transition in Kidney Fibrosis. Journal of the American Society of Nephrology. 2010;21(2):212-22.
[https://doi.org/10.1681/ASN.2008121226]
-
Reidy K, Susztak K. Epithelial-Mesenchymal Transition and Podocyte Loss in Diabetic Kidney Disease. American Journal of Kidney Diseases. 2009;54(4):590-3.
[https://doi.org/10.1053/j.ajkd.2009.07.003]
-
Ziyadeh FN, Wolf G. Pathogenesis of the podocytopathy and proteinuria in diabetic glomerulopathy. Current diabetes reviews. 2008;4(1):39-45.
[https://doi.org/10.2174/157339908783502370]
-
Li JJ, Kwak SJ, Jung DS, Kim JJ, Yoo TH, Ryu DR, et al. Podocyte biology in diabetic nephropathy. Kidney international Supplement. 2007(106):S36-42.
[https://doi.org/10.1038/sj.ki.5002384]
-
Ilatovskaya DV, Levchenko V, Lowing A, Shuyskiy LS, Palygin O, Staruschenko A. Podocyte injury in diabetic nephropathy: implications of angiotensin II-dependent activation of TRPC channels. Scientific reports. 2015;5:17637.
[https://doi.org/10.1038/srep17637]
- SH Jeong, KG Lee, CH Lee, SR Lee, JE Kim, KT Ha, et al. Effects of Hwanggeum-tang Water Extract on the expression of pro-inflammatory responses elicited by advanced glycation end products in THP-1 cells. Korean J. Oriental Physiology & Pathology. 2012:26(2):147-154.
-
Schena FP, Gesualdo L. Pathogenetic mechanisms of diabetic nephropathy. Journal of the American Society of Nephrology : JASN. 2005;16 Suppl 1:S30-3.
[https://doi.org/10.1681/ASN.2004110970]
-
Brownlee M. Biochemistry and molecular cell biology of diabetic complications. Nature. 2001;414(6865):813-20.
[https://doi.org/10.1038/414813a]
-
Mason RM, Wahab NA. Extracellular matrix metabolism in diabetic nephropathy. Journal of the American Society of Nephrology : JASN. 2003;14(5):1358-73.
[https://doi.org/10.1097/01.ASN.0000065640.77499.D7]
-
Gilbert RE, Cooper ME. The tubulointerstitium in progressive diabetic kidney disease: more than an aftermath of glomerular injury? Kidney Int. 1999;56(5):1627-37.
[https://doi.org/10.1046/j.1523-1755.1999.00721.x]
-
Gruden G, Perin PC, Camussi G. Insight on the pathogenesis of diabetic nephropathy from the study of podocyte and mesangial cell biology. Current diabetes reviews. 2005;1(1):27-40.
[https://doi.org/10.2174/1573399052952622]
-
Dai HR, Liu QQ, Liu BL. Research Progress on Mechanism of Podocyte Depletion in Diabetic Nephropathy. Journal of diabetes research. 2017.
[https://doi.org/10.1155/2017/2615286]
-
Ying QD, Wu GZ. Molecular mechanisms involved in podocyte EMT and concomitant diabetic kidney diseases: an update. Renal failure. 2017;39(1):474-83.
[https://doi.org/10.1080/0886022X.2017.1313164]
-
Pagtalunan ME, Miller PL, JumpingEagle S, Nelson RG, Myers BD, Rennke HG, et al. Podocyte loss and progressive glomerular injury in type II diabetes. Journal of Clinical Investigation. 1997;99(2):342-8.
[https://doi.org/10.1172/JCI119163]
-
Dai H, Liu Q, Liu B. Research Progress on Mechanism of Podocyte Depletion in Diabetic Nephropathy. Journal of diabetes research. 2017;2017:2615286.
[https://doi.org/10.1155/2017/2615286]
-
Iwano M, Plieth D, Danoff TM, Xue C, Okada H, Neilson EG. Evidence that fibroblasts derive from epithelium during tissue fibrosis. Journal of Clinical Investigation. 2002;110(3):341-50.
[https://doi.org/10.1172/JCI0215518]
-
Ng YY, Huang TP, Yang WC, Chen ZP, Yang AH, Mu W, et al. Tubular epithelial-myofibroblast transdifferentiation in progressive tubulointerstitial fibrosis in 5/6 nephrectomized rats. Kidney International. 1998;54(3):864-76.
[https://doi.org/10.1046/j.1523-1755.1998.00076.x]
-
Rastaldi MP, Ferrario F, Giardino L, Dell'Antonio G, Grillo C, Grillo P, et al. Epithelial-mesenchymal transition of tubular epithelial cells in human renal biopsies. Kidney International. 2002;62(1):137-46.
[https://doi.org/10.1046/j.1523-1755.2002.00430.x]
-
Burns WC, Twigg SM, Forbes JM, Pete J, Tikellis C, Thallas-Bonke V, et al. Connective tissue growth factor plays an important role in advance glycation end product-induced tubular epithelial-to-mesenchymal transition: Implications for diabetic renal disease. Journal of the American Society of Nephrology. 2006;17(9):2484-94.
[https://doi.org/10.1681/ASN.2006050525]
-
Loeffler I, Wolf G. Transforming growth factor-beta and the progression of renal disease. Nephrol Dial Transpl. 2014;29:I37-I45.
[https://doi.org/10.1093/ndt/gft267]
-
Kitamura M, Suto TS. TGF-beta and glomerulonephritis: Anti-inflammatory versus prosclerotic actions. Nephrol Dial Transpl. 1997;12(4):669-79.
[https://doi.org/10.1093/ndt/12.4.669]
-
Lopez-Hernandez FJ, Lopez-Novoa JM. Role of TGF-beta in chronic kidney disease: an integration of tubular, glomerular and vascular effects. Cell and tissue research. 2012;347(1):141-54.
[https://doi.org/10.1007/s00441-011-1275-6]
-
Han DC, Hoffman BB, Hong SW, Guo J, Ziyadeh FN. Therapy with antisense TGF-beta1 oligodeoxynucleotides reduces kidney weight and matrix mRNAs in diabetic mice. American journal of physiology Renal physiology. 2000;278(4):F628-34.
[https://doi.org/10.1152/ajprenal.2000.278.4.F628]
-
Wrana JL, Attisano L, Wieser R, Ventura F, Massague J. Mechanism of activation of the TGF-beta receptor. Nature. 1994;370(6488):341-7.
[https://doi.org/10.1038/370341a0]
-
Lamouille S, Derynck R. Cell size and invasion in TGF-beta-induced epithelial to mesenchymal transition is regulated by activation of the mTOR pathway. J Cell Biol. 2007;178(3):437-51.
[https://doi.org/10.1083/jcb.200611146]
-
Wullschleger S, Loewith R, Hall MN. TOR signaling in growth and metabolism. Cell. 2006;124(3):471-84.
[https://doi.org/10.1016/j.cell.2006.01.016]
-
Zhou HY, Huang SL. Current development of the second generation of mTOR inhibitors as anticancer agents. Chin J Cancer. 2012;31(1):8-18.
[https://doi.org/10.5732/cjc.011.10281]
-
Godel M, Hartleben B, Herbach N, Liu SY, Zschiedrich S, Lu S, et al. Role of mTOR in podocyte function and diabetic nephropathy in humans and mice. Journal of Clinical Investigation. 2011;121(6):2197-209.
[https://doi.org/10.1172/JCI44774]
-
Inoki K, Mori H, Wang JY, Suzuki T, Hong SK, Yoshida S, et al. mTORC1 activation in podocytes is a critical step in the development of diabetic nephropathy in mice. Journal of Clinical Investigation. 2011;121(6):2181-96.
[https://doi.org/10.1172/JCI44771]
-
Inoki K, Zhu T, Guan KL. TSC2 mediates cellular energy response to control cell growth and survival. Cell. 2003;115(5):577-90.
[https://doi.org/10.1016/S0092-8674(03)00929-2]