
인간 A549 폐암 세포에서 더덕 뿌리 추출물의 세포 증식 및 침습 억제 효과
Ⓒ The Society of Pathology in Korean Medicine, The Physiological Society of Korean Medicine
Abstract
Non-small cell lung cancer(NSCLC) is a highly malignant tumor with limited treatment options. Conventional chemotherapy significant has limitations, including drug resistance and side effects. In this study, we investigated the anticancer effects of Codonopsis lanceolata root extract(CLRE), a traditional medicinal herb, on the human lung cancer cell line A549. Our results showed that CLRE inhibited A549 cell proliferation, migration, and invasion. Furthermore, CLRE effectively suppressed epithelial-to-mesenchymal transition(EMT) markers, including N-cadherin, vimentin, and MMP-9, induced by TGF-β1. Also CLRE inhibited cell migration and invasion in A549 cells through the downregulation of the PI3K/AKT signaling pathway. These findings suggest that CLRE has potential as a natural anticancer agent or adjunct therapy for NSCLC by inhibiting cell proliferation and EMT processes. Further studies are needed to elucidate the underlying mechanisms and assess the safety and therapeutic potential of CLRE in preclinical and clinical studies.
Keywords:
Codonopsis lanceolata, Non-small cell lung cancer (NSCLC), Anticancer, Epithelial-to-mesenchymal transition (EMT), Cell invasion서 론
폐암은 전 세계적으로 암 관련 사망의 주요 원인이며 모든 암 유형 중에서 가장 나쁜 예후를 갖는 암 중 하나입니다. 이 중 80% 이상을 차지하는 비소세포 폐암(Non-Small Cell Lung Cancer; NSCLC)은 치료하기 가장 어려운 악성 종양 중 하나입니다1,2). NSCLC 환자들은 초기 단계에서 임상적 증상이 없기 때문에 진행된 단계나 전이 단계에서 진단되는 경우가 많으며, 이는 나쁜 예후를 보이는 원인 중 하나입니다2,3). NSCLC의 발생과 진행에는 다양한 병리학적 메커니즘이 관여하며, 이로 인해 뼈, 뇌, 간 및 림프절로의 전이가 쉽게 발생하여 치료에 어려움을 초래합니다4). 최근 면역요법과 표적치료 등의 치료법이 발전하고 있지만, 진행성 및 전이성 폐암 치료에 여전히 화학요법이 주로 사용됩니다. 그러나 화학요법은 치료 효능이 제한적일 수 있으며, 약물 저항성이 발생할 수 있다. 또한, vandetanib과 bevacizumab과 같은 화학제제는 구토, 구역질, 탈모 및 출혈 등과 같은 부작용을 유발할 수 있다5,6). 따라서 이러한 부작용을 보완하기 위한 대체 치료 전략으로, 기능성 식품 및 의약품 자원으로서의 천연물 연구가 활발히 진행되고 있다.
상피-중간엽 전이(Epithelial-to-Mesenchymal Transition; EMT)는 암세포 전이의 초기 단계에서 중요한 역할을 하며, 이 과정에서 세포는 상피 극성 표현형에서 섬유아세포 또는 중간엽 표현형으로 변화한다7,8). EMT는 E-cadherin 발현 감소, N-cadherin 및 vimentin 발현 증가로 특징지어지며, 이는 암세포의 이동성과 전이 가능성을 높이고, 악성 종양의 예후를 악화시키며 재발 가능성을 증가 시킨다9). NSCLC의 표적 치료제로 상피세포 성장 인자 수용체-티로신 키나제 억제제(Epidermal Growth Factor Receptor-Tyrosine Kinase Inhibitors; EGFR-TKIs)가 사용되지만, 환자들은 치료 후 획득 저항성을 발달시켜 임상 효능이 제한된다10,11). EMT는 TGF-β, Wnt/β-catenin, Notch, EGF, HGF, FGF 등과 같은 여러 신호 경로를 포함하는 복잡한 네트워크로 구성된다12). 이 중 TGF-β는 세포 성장, 증식 및 분화를 촉진하는 조절자로, 높은 이동성과 침윤 능력을 유도하여 EMT in vitro 모델로 널리 사용된다13).
더덕(Codonopsis lanceolata)은 초롱꽃과의 덩굴식물로 한국, 일본 및 중국 등 동남아시아의 산간 지방에 자생하는 다년생 초본으로, 전통적으로 약용 및 식용으로 활용되어 왔다14). 전통 의학에서 C. lanceolata는 해열, 해독, 인후염, 경련, 암, 비만, 고지혈증, 부종, 간염, 대장염 등의 질병 및 증상을 치료하는데 널리 사용되었다15-17). 특히, C. lanceolata의 뿌리는 기침, 기관지염, 부종, 천식, 폐암 등 다양한 폐 질환을 치료하는 민간요법으로 사용되어 왔다18). C. lanceolata 뿌리는 항산화, 항미생물, 항염증, 항암 및 면역조절과 같은 약리학적 특성을 나타내는 것으로 보고되었다15,19,20). 이전 연구들에 따르면, C. lanceolata는 다양한 약리학적 활성을 나타내는 polyphenols, saponins, tannins, triterpene, alkaloids, 및 steroids를 비롯한 많은 생리활성 화합물을 함유하고 있다15,21). 그러나 이러한 다양한 약리학적 활성에도 불구하고, C. lanceolata 뿌리 추출물(CLRE) 또는 그 추출물에서 분리된 새로운 화합물의 폐암에 대한 항암 효과는 명확하게 밝혀지지 않았다.
현재까지 NSCLC의 치료에는 주로 화학요법이 사용되어 왔으나, 이 치료법은 일부 환자에서 효과가 제한적일 수 있다. 이러한 한계로 인해 NSCLC 치료의 대안적 접근법으로서 천연물의 활용이 주목받고 있으며, 특히 C. lanceolata와 같은 식물의 추출물 또는 구성성분들이 새로운 치료 접근법으로 제시될 수 있다.
본 연구에서는 C. lanceolata의 뿌리 추출물이 인간 폐암 세포주인 A549 세포의 증식 및 침습을 억제시켜 항암 효과를 나타내는지 평가하고자한다.
재료 및 방법
1. 더덕(C. lanceolata) 뿌리 추출물 제조
더덕(C. lanceolata) 뿌리는 2018년 10월, 경동 생약 시장(Seoul, Korea)에서 구입하였다. 간략하게, 구입한 더덕 뿌리는 물로 세척한 후, 절편으로 만들어 동결 건조하였다. 동결 건조된 더덕 뿌리(100 g)는 중량의 10배인 70% 에탄올을 첨가하여 80℃에서 8시간 동안 3회 반복 추출하였다. 추출물은 감압 여과한 후, rotary evaporator를 사용하여 용매를 제거하고, 동결 건조하여 분말 형태로 농축하였다. 최종적으로 얻은 분말은 이후 실험에 사용하였다.
2. 세포 배양 및 EMT 유도
인간 폐 상피에서 유래한 비소세포 폐암 세포주인 A549는 American Type Culture Collection(Rockville, MD, USA)에서 구입하였다. 세포주는 penicillin 및 streptomycin(100 U/ml) 그리고 10% fetal bovine serum(Gibco BRL, Gaithersburg, MD, USA)이 포함된 RPMI 1640 배지(Gibco)하에서 37 ℃와 5% CO2 조건에서 배양하였다. EMT 과정을 유도하기 위해 A549 세포에 TGF-β1(5 ng/mL; R&D Systemss, Minneapolis, MN)을 48시간 동안 처리하였다.
3. 세포 생존 및 집락 형성 검사
세포 생존율을 확인하기 위해 WST-1 검사(ITSBio, Seoul, Korea)와 집락 형성 검사(Colony formation assay; CFA)를 사용하여 측정하였다. WST-1 검사의 경우, A549 세포(5 X 103/well)를 96 well 플레이트에 분주하고 24시간 후, CLRE(250, 500, 750, 1000 μg/ml)를 24 또는 48시간 동안 처리하고, SpectraMAX 250 microplate spectrophotometer(Molecular Devices, Sunnyvale, CA)를 이용하여 450 nm에서 흡광도를 측정하였다. 세포생존율은 다음과 같이 계산하여 백분율(%)로 나타내었다.
Cell viability(%) = (OD450 of treated cells/OD450 of control cells) × 100
CFA의 경우 A549 세포(5 X 103/well)를 12 well 플레이트에 분주하고 CLRE(250, 750, 1000 μg/ml)을 처리한 후 10일 동안 배양하였다. 10일 후, 콜로니를 메탄올에 고정하고 메틸린 블루로 염색하였다.
4. In vitro migration 및 invasion 검사
A549 세포의 이동 및 침습 능력을 평가하기 위해 24-transwell 챔버(Corning Life Sciences, Acton, MA)와 Matrigel로 코팅된 invasion 챔버(BD Biosciences, San Jose, CA)를 사용하여 측정하였다. In vitro migration 검사의 경우, A549 세포(4 X 104/well)를 위쪽 챔버에 분주하였다. 12시간 후 아래쪽 챔버에 TGF-β1(5 ng/mL), TGF-β1 + CLRE(750 μg/ml)을 48시간 처리하였다. In vitro invasion 검사의 경우, A549 세포(8 X 104/well)를 위쪽 챔버에 분주하였다. 12시간 후 아래쪽 챔버에 TGF-β1(5 ng/mL), TGF-β1 + CLRE(750 μg/ml)을 72시간 처리하였다. 48 또는 72시간 후 membrane 아래쪽에 있는 세포를 고정하고 Diff-Quick 용액을 사용하여 염색하였다. membrane의 아랫면으로 이동하거나 침습한 세포를 well당 5개의 현미경 시야(배율 ×100)에서 세었으며 촬영하였다.
5. Western blot
세포는 차가운 1X PBS(pH 7.4)로 세척하고, protease & phosphatase inhibitor cocktail(Millipore, Darstadt, Germany)이 포함된 RIPA lysis buffer로 세포를 용해시켰다. 분리된 단백질은 Bradford 검사법을 이용하여 정량하였다. 30 μg의 단백질을 SDS-PAGE 젤로 분리한 후 PVDF membrane으로 전이시켰다. Membrane은 5% skim milk가 포함된 Tris-buffered saline+Tween-20(20 mM Tris-HCl, pH 7.6, 150 mM NaCl, 0.05% Tween-20)으로 1시간 동안 차단시킨 후, 1차 항체를 결합시켰다. 사용된 1차 항체는 다음과 같다: Ras(Cell Signaling Technology, Beverly, MA, USA), PI3K(Cell Signaling), p-AKT(Cell Signaling), AKT(Cell Signaling), vimentin(Cell Signaling), MMP-9(Cell Signaling), E-cadherin(BD Biosciences), N-cadherin(BD Biosciences), 및 GAPDH(Sigma-Aldrich). 세척 후, membrane을 2차 항체에 결합하고 실온에서 1시간 동안 반응시키고 ECL kit(Millipore)와 C-Digit western scanner(LI-COR, Lincoln, NE, USA)를 사용하여 단백질을 검출하고 이미지화하였다.
6. RNA 추출 및 Quantitative Real-Time PCR(qRT-PCR) 분석
세포는 차가운 1X PBS (pH 7.4)로 세척하고, TRIzol 시약(Invitrogen, Carlsbad, CA, USA)을 사용하여 total RNA를 추출하였다. 제조사의 방법에 따라 PrimeScript™ RT 시약 키트(TaKaRa Bio, Shiga, Japan)를 사용하여 total RNA 1 μg의 역전사를 수행하였다. SYBR Green PCR Master Mix(Applied Biosystems, Foster City, CA, USA) 및 ABI Prism 7900 Sequence Detection System(Applied Biosystems)을 이용하여 qRT-PCR에 수행하였다. 각 유전자의 mRNA 발현양은 GAPDH 유전자 발현에 대한 상대적인 양으로 계산하였다. qRT-PCR에 사용되는 primer sequence는 Table 1에 나열하였다.
7. 통계학적 분석
모든 실험 결과는 평균 ± 표준편차로 표시하고 Student’s t-test와 SPSS(version 20, IBM SPSS Statistics, USA)의 one-way analysis of variance(ANOVA)를 사용하여 검정하였다. p<0.05의 경우에서 통계적 유의성이 있는 것으로 간주하였다.
결 과
1. 더덕 뿌리 추출물(CLRE)의 세포생존율에 미치는 효과
CLRE를 각각의 농도별(250, 500, 750, 1000 μg/ml)로 A549 세포에 처리한 후 시간 및 농도 의존적 방식으로 A549 세포의 세포생존율을 WST-1과 CFA 검사를 사용하여 확인하였다. 그 결과 CLRE는 대조군과 비교하여 시간 및 농도 의존적으로 A549 세포의 증식을 억제하였다. 24 및 48시간 동안 CLRE(500 μg/ml) 처리군의 경우 대조군에 비하여 각각 약 20%와 30% 이상 세포 증식이 유의하게 억제되었으며, CLRE(750 μg/ml) 처리군에서는 약 45%와 55% 이상 세포 증식이 유의하게 억제되는 것을 확인하였다(Fig. 1A). 추가적으로, CLRE는 A549 세포의 집락 형성도 농도 의존적으로 억제하였다(Fig. 1B).
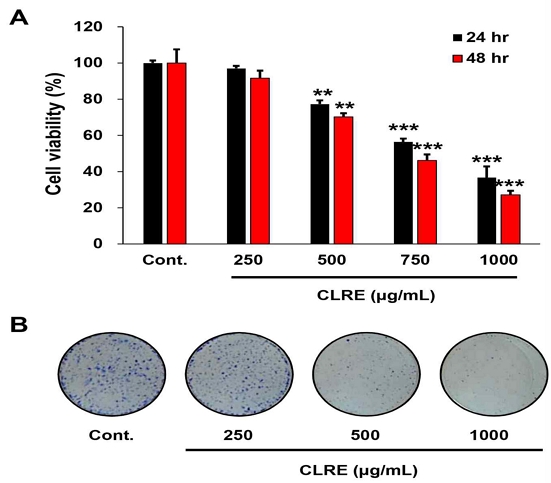
CLRE decreases the proliferation of lung cancer cells. (A) Cell viability was measured using the WST-1 assay after 24, 48 hours of treatment with varying concentrations of CLRE. CLRE significantly inhibited the proliferation of A549 cells in a dose- and time-dependent manner. (B) Colony forming assays were conducted to assess the proliferation of A549 cells following 10 days of CLRE treatment. (**P<0.005; ***P<0.001.)
2. CLRE의 세포 증식 관련 단백질 및 mRNA 발현에 대한 효과
Fig. 1에서 보여준 것처럼, CLRE가 A549 세포의 증식과 유의하게 관련이 있다는 것을 보여주었다. 따라서 우리는 폐암세포의 증식과 관련된 분자의 발현을 Western blot과 qRT-PCR 방법을 이용하여 분석하였다. CLRE(500 μg/ml) 처리 군에서 Ras, PI3K, p-AKT, 및 AKT의 단백질 발현은 대조군과 비교하였을 때 Ras, PI3K, p-AKT, 및 AKT의 단백질 발현이 감소하였다(Fig. 2A). 또한, 단백질 발현과 유사하게 KRAS, PI3KCA, 및 AKT의 mRNA 발현도 유의하게 감소하였다(Fig. 2B).
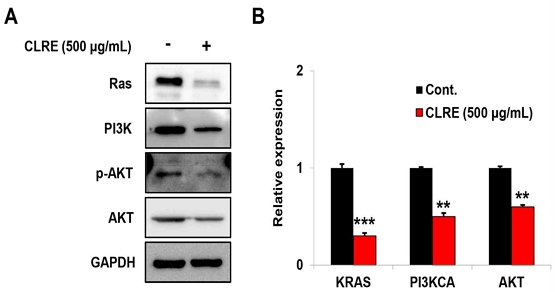
CLRE is associated with the expression of signaling molecules associated with the proliferation of lung cancer cells. (A) Western blotting was performed for Ras, PI3K, p-AKT, and AKT after CLRE treatment in A549 cells. The protein expression levels of Ras, PI3K, p-AKT, and AKT decreased by CLRE in A549 cells. (B) Quantitative reverse-transcription polymerase chain reaction was performed for KRAS, PI3KCA, and AKT after CLRE treatment in A549 cells. In the CLRE treated group, the mRNA levels of KRAS, PI3KCA, and AKT significantly decreased compared to the control group.(**P<0.005; ***P<0.001)
3. CLRE의 A549 세포 이동 및 침습 억제 효과
앞서 CLRE가 A549 세포에서 항증식 효과를 나타낸 것을 확인하였다. 따라서 CLRE의 항암 효과를 알아보기 위해 A549 세포의 이동 및 침습 능력을 in vitro migration 및 invasion 검사를 통해 확인하였다. A549 세포의 이동성을 높이는 EMT를 유도하기 위해 TGF-β1(5 ng/mL)을 사용하였다. 그 결과, A549 세포에 TGF-β1을 처리한 그룹에서 세포의 이동 및 침습 능력이 대조군에 비해 유의하게 증가하는 것을 확인하였다. 그러나 TGF-β1 + CLRE(500 μg/ml)를 처리한 그룹에서는 TGF-β1에 의해 증가된 A549 세포의 이동 및 침습능이 CLRE에 의해 유의하게 억제되는 것을 보여주었다(Fig. 3A). 정량 분석 결과, CLRE는 TGF-β1에 의해 증가된 A549 세포 이동 및 침습을 각각 30% 및 26% 억제하였다(Fig. 3B).
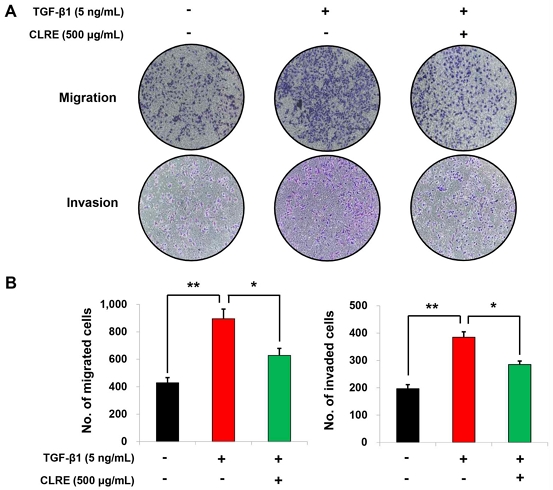
CLRE decreases TGF-β1-induced lung cancer cell migration and invasion. (A) Cell migration and invasion assays were performed to evaluate the invasiveness of TGF-β1-induced A549 cells after CLRE treatment. (B) The number of cells which migrated or invaded the chambers was counted in five microscopic fields per well at 100x magnification.(**P<0.01, vs. control group, *P<0.05, vs. TGF-β1 only treatment group)
4. CLRE의 EMT 관련 단백질 및 mRNA 발현에 대한 효과
Fig. 3에서 볼 수 있듯이 CLRE는 A549 세포의 이동 및 침습과 유의하게 관련이 있다는 것을 확인하였다. 따라서 우리는 폐암 세포의 이동 및 침습에 관여하는 EMT 관련 분자들의 발현을 조사하였다. EMT 유도를 위해 A549 세포에 TGF-β1을 처리한 군에서는 대조군에 비해 N-cadherin, vimentin, 및 MMP-9의 단백질과 mRNA의 발현이 유의하게 증가하였고, E-cadherin의 발현은 감소하였다. 그러나 TGF-β1에 의해 증가된 N-cadherin, vimentin, 및 MMP-9의 발현은 CLRE을 처리하였을 때 단백질과 mRNA의 발현이 유의하게 감소하였고, TGF-β1에 의해 감소된 E-cadherin의 발현은 증가하였다(Fig. 4A, B).
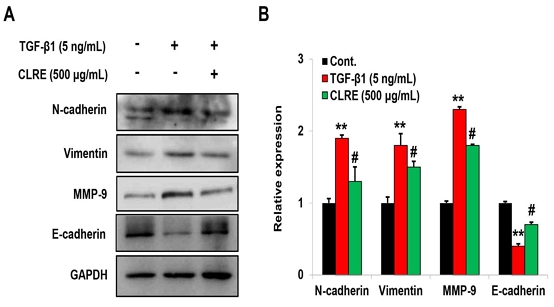
CLRE reduces the expression of EMT related molecules induced by TGF-β1 in lung cancer cells. (A) Western blotting was conducted to analyze the expression of N-cadherin, vimentin, MMP-9, and E-cadherin in A549 cells following TGF-β1 treatment, with or without CLRE. (B) The mRNA expression patterns for N-cadherin, vimentin, MMP-9, and E-cadherin were analyzed via quantitative reverse-transcription polymerase chain reaction after TGF-β1 treatment with or without CLRE in A549 cells.(**P<0.005, vs. control group, #P<0.01, vs. TGF-β1 only treatment group)
고 찰
비소세포 폐암(NSCLC)은 전 세계적으로 가장 흔한 악성 종양으로 알려져 있다1,2). 이 질환의 분자적 메커니즘은 널리 연구되었으며, 새로운 치료적 접근법의 필요성도 커지고 있다. 현재 platinum 또는 tyrosine kinase inhibitor 기반 화학 요법이 폐암의 표준 치료법으로 사용되고 있으나, 화학 요법의 효능은 약물 내성과 독성 효과로 인해 크게 제한된다22,23). 전통 의학에서는 강래특, 고삼, Javanica oil, 황기 추출물 등의 보조 요법이 화학 요법과 함께 사용되어 폐암 예방과 치료에 기여하고, 이는 환자의 예후를 개선하고 합병증을 감소시키는데 도움울 줄 수 있다고 보고되었다24-26). 따라서 화학 요법의 부작용을 보완할 수 있는 천연물 및 한약재를 탐색하고, 대안적 치료법을 개발하는 것이 중요하다.
C. lanceolata 뿌리는 항산화, 항미생물, 항염증, 항암 및 면역조절과 같은 약리학적 특성을 나타내며, polyphenols, saponins, alkaloids, 및 polysarcaroids를 비롯한 많은 생리활성 성분을 함유하고 있다고 보고되었다15,19,20). 그러나 C. lanceolata 뿌리 추출물(CLRE)의 폐암에 대한 항암 효과는 명확하게 밝혀지지 않았다.
이전 연구에 따르면, C. lanceolata 뿌리에서 얻은 polyacetylenes이 용량 및 시간에 따라 A549 세포의 증식을 억제하고, A549 세포를 이종 이식받은 누드 마우스에서 종양 성장을 억제시켰다18). 또한, C. lanceolata의 주요 생리활성 물질인 lancemaside A가 A549 세포와 A2780 난소암 세포의 생존율을 억제한다는 것을 확인하였다27,28). 더불어, C. lanceolata 뿌리 추출물이 H22 간암세포를 이식받은 마우스의 종양 성장을 억제하여 항암 활성을 나타낸다고 보고되었다29). 이번 연구에서 CLRE이 A549 세포의 증식을 농도 및 시간 의존적으로 억제시키는 것을 확인하였다.
PI3K/AKT 경로는 암세포의 생리적 과정에 중요한 역할을 하며, 이 경로의 비정상적 발현은 인간 암에서 흔히 나타난다30,31). PI3K는 Ras에 의해 활성화되며, 이후 다양한 세포 내 신호전달을 통해 세포 주기 조절, DNA 복구, 세포 사멸 등 많은 생리적 과정에 참여한다18,32,33). 또한, AKT는 Bax를 억제하고, GSK-3β를 인산화하며, caspase-9의 활성을 억제한다. 이로 인해 caspase-9에 의한 caspase-3의 절단 및 활성화가 억제되어, 결과적으로 항세포사멸 성분의 발현이 증가하고 세포 생존 신호를 증가시킨다18,34). 이번 연구에서 우리는 CLRE가 A549 세포에서 Ras, PI3K, p-AKT 및 AKT의 단백질과 mRNA의 발현을 유의하게 감소시키는 것을 확인하였다. 따라서, 이번 연구 결과는 CLRE가 Ras/PI3K/AKT 경로를 조절하여 A549 세포의 세포 사멸을 유도하고, 이로 인해 항증식 효과를 나타냄을 시사합니다.
EMT는 NSCLC 진행에 관여하는 경로 중 하나로, 상피 세포가 상피 마커의 손실과 중간엽 마커의 획득을 통해 이동성과 침습성을 획득하면서 종양이 초기 단계에서 침습적 단계로 전환되는 가역적 과정입니다35). TGF-β1은 다양한 조직의 만성 염증에서 중요한 역할을 하며, EMT의 강력한 프로모터로 알려져 있다13). 따라서 EMT를 억제하는 것은 암 전이를 방지하는 중요한 전략이 될 수 있다. 이번 연구의 흥미로운 발견 중 하나는 CLRE이 TGF-β1에 의해 EMT 유도된 A549 세포의 이동과 침윤을 억제하는 것을 확인하였다. 이번 결과와 유사하게 C. lanceolata 뿌리에서 추출한 polyacetylenes는 A549 세포의 이동 및 침윤을 억제한 바 있다18). 이전 연구에서 C. lanceolata 뿌리에서 추출된 lancemaside A가 난소암 세포의 이동 및 침습능을 유의하게 억제한다는 것을 보고하였다36). 또한, C. lanceolata 뿌리 추출물이 흑색종의 in vitro 및 in vivo 모델에서 항전이 효과를 나타낸다는 것을 보고하였다37).
EMT의 주요 특징은 E-cadherin 발현 감소와 N-cadherin, vimentin 및 MMP-9 발현 증가로 잘 알려져 있다7-9,35). 이전 연구에서는 C. lanceolata 뿌리에서 분리된 lancemaside A가 난소암 세포에서 MMP-2와 MMP–9의 발현을 감소시킨다는 것을 확인하였다36). 또한, C. lanceolata 추출물이 bFGF에 의해 증가된 MMP-2와 MMP–9의 발현을 감소시켰다38). 이번 연구 결과에서, TGF-β1에 의해 EMT가 유도된 A549 세포에서 CLRE가 EMT 마커인 N-cadherin, vimentin 및 MMP-9의 단백질과 mRNA 발현을 감소시키고, E-cadherin의 발현을 증가시키는 것을 확인할 수 있었다. 추가적으로, EMT는 TGF-β, Wnt/β-catenin, Notch, growth factor 등 다양한 신호 경로가 연관된 복잡한 네트워크로 구성되어 있으며12), ROS, NF-κB, MAPKs, PI3K/AKT 신호 경로가 TGF-β에 의해 유도되는 EMT를 조절하는 데 중요한 역할을 한다39-41). 최근 연구에 따르면 C. lanceolata 뿌리에서 분리된 lancemaside A가 난소암 세포에서 ROS를 매개로 하는 p38 경로를 통해 난소암 세포 침습을 억제하는 것을 확인되었다36). TGF-β 수용체 복합체에는 PI3K/AKT 경로와 관련이 있으며42), TGF-β는 TGF-β 수용체 또는 EGF 수용체를 통해 PI3K를 활성화시키며, EMT 유도 전사인자의 발현을 촉진할 수 있는 AKT의 활성화를 촉진시킨다43). AKT는 또한 GSK-3β를 억제하여 snail1을 인산화하거나 NF-κB를 활성화하여 편평세포암 세포에서 EMT를 유도한다44). C. lanceolata 뿌리에서 추출한 polyacetylenes는 A549 세포의 PI3K와 AKT을 발현을 억제한 바 있다18). 또한, C. lanceolata 뿌리 추출물이 B16F10 흑색종 세포에서 β1 integrin/FAK/paxillin을 경로를 조절하여 세포 이동을 억제한다고 보고하였다37). FAK는 PI3K를 직접적으로 활성화 하는 것으로 알려져 있다. 이번 연구에서 TGF-β1이 PI3K, p-AKT 및 AKT를 증가시키는 반면 CLRE은 TGF-β1에 의해 증가된 발현을 억제하였다. 이러한 결과는 CLRE이 TGF-β1 유도된 A549 세포에서 EMT 진행을 PI3K/AKT 경로를 통해 억제할 수 있음을 시사합니다.
EMT는 약물 내성과 밀접한 관련이 있고, EMT 진행은 세포에 침습 및 이동 능력을 부여하여 약물 내성에 기여하며, EGF-TKI 감수성 회복은 EMT 역전과 관련이 있다는 보고가 있다45). NSCLC의 치료제인 erlotinib에 대한 내성을 획득한 폐암 환자는 폐 조직에 EMT가 있는 것으로 보고되었다46). 또한 geftinib 및 osimertinib 내성 NSCLC 세포는 EGFR 2차 돌연변이 없이 E-cadherin이 감소하고 vimentin이 증가하는 등 EMT 특성을 보였다47). 따라서 EMT는 EGFR-TKI 내성 NSCLC의 중요한 메커니즘 중 하나로 볼 수 있다. 그러나 이번 연구에서는 CLRE가 EMT에 의한 항암제 내성에 관여하는 데이터가 제한적이다. 따라서 CLRE가 EMT와 관련된 항암제 내성 메커니즘을 명확하게 확인하기 위해 추가 연구가 필요합니다.
종합적으로, 본 연구는 CLRE가 Ras/PI3K/AKT 신호 경로와 TGF-β1 유도 EMT 과정을 억제하여 A549 폐암 세포의 증식과 이동을 효과적으로 억제할 수 있음을 시사합니다. 이러한 결과는 CLRE가 폐암 치료에 있어 천연 물질 및 기능성 식품으로서의 잠재력을 지니고 있음을 제시합니다. 그러나, CLRE의 명확한 신호 전달 경로와 정상 폐 세포에 대한 영향 및 전임상 연구를 통한 안전성 검증이 추가적으로 필요합니다.
결 론
본 연구는 C. lanceolata 뿌리 추출물(CLRE)이 A549 폐암 세포의 증식을 농도 및 시간 의존적으로 억제하며, TGF-β1에 의해 유도된 EMT 관련 마커인 N-cadherin, vimentin, 및 MMP-9의 발현을 하향 조절함으로써 세포 이동과 침습을 억제함을 확인하였다. 이러한 결과는 CLRE가 Ras/PI3K/AKT 신호 경로를 억제하여 세포 증식 및 EMT 과정을 저해함으로써 항암 효과를 나타냄을 시사합니다. 결론적으로, CLRE는 NSCLC 치료에서 천연 항암제 또는 보조 치료제로서의 잠재력을 지니고 있으며, 후속 연구를 통해 이러한 기전을 더욱 명확히 밝히고, 전임상 및 임상 연구를 통해 CLRE의 안전성과 치료적 활용 가능성을 평가할 필요가 있습니다.
Acknowledgments
이 논문은 2023학년도 원광대학교의 교비지원에 의해 수행됨
References
-
Slatore C, Lareau SC, Fahy B. Staging of Lung Cancer. Am J Respir Crit Care Med. 2022;205(9):17-19.
[https://doi.org/10.1164/rccm.2059P17]
-
Spira A, Ettinger DS. Multidisciplinary management of lung cancer. N Engl J Med. 2004;350(4):379-92.
[https://doi.org/10.1056/NEJMra035536]
-
Mithoowani H, Febbraro M. Non-small-cell lung cancer in 2022: a review for general practitioners in oncology. Curr Oncol. 2022;29(3):1828-39.
[https://doi.org/10.3390/curroncol29030150]
-
Zhu T, Bao X, Chen M, Lin R, Zhuyan J, Zhen T, et al. Mechanisms and Future of Non-Small Cell Lung Cancer Metastasis. Front Oncol. 2020;10:585284.
[https://doi.org/10.3389/fonc.2020.585284]
- Korpanty G, Smyth E, Carney DN. Update on anti-angiogenic therapy in non-small cell lung cancer: Are we making progress? J Thorac Dis. 2011;3(1):19-29.
-
Islam KM, Anggondowati T, Deviany PE, Ryan JE, Fetrick A, Bagenda D, et al. Patient preferences of chemotherapy treatment options and tolerance of chemotherapy side effects in advanced stage lung cancer. BMC Cancer. 2019;19(1):835.
[https://doi.org/10.1186/s12885-019-6054-x]
-
Serrano-Gomez SJ, Maziveyi M, Alahari SK. Regulation of epithelial–mesenchymal transition through epigenetic and post-translational modifications. Mol Cancer. 2016;15(1):18.
[https://doi.org/10.1186/s12943-016-0502-x]
-
Gavert N, Ben-Ze'ev A. Epithelial-mesenchymal transition and the invasive potential of tumors. Trends Mol Med. 2008;14(5):199-209.
[https://doi.org/10.1016/j.molmed.2008.03.004]
-
Xu J, Lamouille S, Derynck R. TGF-β-induced epithelial to mesenchymal transition. Cell Res. 2009;19(2):156-72.
[https://doi.org/10.1038/cr.2009.5]
-
Takeda Y, Naka G, Yamaguchi Y, Hashimoto M, Suzuki M, Izumi S, et al. Genetic diagnostic features after failure of initial treatment with epidermal growth factor receptor (EGFR)-tyrosine kinase inhibitors among non-small-cell lung cancer patients harboring EGFR mutations. BMC Cancer. 2020;20(1):951.
[https://doi.org/10.1186/s12885-020-07424-w]
-
Zhu X, Chen L, Liu L, Niu X. EMT-mediated acquired EGFR-TKI resistance in NSCLC: mechanisms and strategies. Front Oncol. 2019;9:1044.
[https://doi.org/10.3389/fonc.2019.01044]
-
Gonzalez DM, Medici D. Signaling mechanisms of the epithelial–mesenchymal transition. Sci Signal. 2014;7(344):re8.
[https://doi.org/10.1126/scisignal.2005189]
-
Willis BC, Borok Z. TGF-β-induced EMT: mechanisms and implications for fbrotic lung disease. Am J Physiol Lung Cell Mol Physiol. 2007;293(3):L525-34.
[https://doi.org/10.1152/ajplung.00163.2007]
-
Lee KT, Choi J, Jung WT, Nam JH, Jung HJ, Park HJ. Structure of a new echinocystic acid bisdesmoside isolated from Codonopsis lanceolata roots and the cytotoxic activity of prosapogenins. J Agric Food Chem. 2002;50(15):4190-3.
[https://doi.org/10.1021/jf011647l]
-
Hossen MJ, Kim MY, Kim JH, Cho JY. Codonopsis lanceolata: A Review of Its Therapeutic Potentials. Phytother Res. 2016;30(3):347-56.
[https://doi.org/10.1002/ptr.5553]
- Yoo HH, Baek SH, Park YK, Lee SH, Kim CM, Lee KS, et al. Quality control of dried roots of Codonopsis lanceolata. Kor J Pharmacogn. 2002;33(2):85-7.
- Han EG, Sung IS, Moon HG, Cho SY. Effect of Codonopsis lanceolata water extract on the levels of lipid in rats fed high fat diet. J Korean Soc Food Sci Nutr. 1998;27:940-4.
-
Wang MC, Wu YF, Yu WY, Yu B, Ying HZ. Polyacetylenes from Codonopsis lanceolata Root Induced Apoptosis of Human Lung Adenocarcinoma Cells and Improved Lung Dysbiosis. Biomed Res Int. 2022;2022:7713355.
[https://doi.org/10.1155/2022/7713355]
-
Byeon SE, Choi WS, Hong EK, Lee J, Rhee MH, Park HJ, et al. Inhibitory effect of saponin fraction from Codonopsis lanceolata on immune cell-mediated inflammatory responses. Arch Pharm Res. 2009;32(6):813-22.
[https://doi.org/10.1007/s12272-009-1601-7]
-
Xu LP, Wang H, Yuan Z. Triterpenoid saponins with anti-inflammatory activity from Codonopsis lanceolata. Planta Med. 2008;74(11):1412-5.
[https://doi.org/10.1055/s-2008-1081318]
-
Du YE, Lee JS, Kim HM, Ahn JH, Jung IH, Ryu JH, Choi JH, Jang DS. Chemical constituents of the roots of Codonopsis lanceolata. Arch Pharm Res. 2018;41(11):1082-91.
[https://doi.org/10.1007/s12272-018-1080-9]
-
Lv P, Man S, Xie L, Ma L, Gao W. Pathogenesis and therapeutic strategy in platinum resistance lung cancer. Biochim Biophys Acta Rev Cancer. 2021;1876(1):188577.
[https://doi.org/10.1016/j.bbcan.2021.188577]
-
Chen JA, Riess JW. Advances in targeting acquired resistance mechanisms to epidermal growth factor receptor tyrosine kinase inhibitors. J Thorac Dis. 2020;12(5):2859-76.
[https://doi.org/10.21037/jtd.2019.08.32]
-
Li Z, Feiyue Z, Gaofeng L. Traditional Chinese medicine and lung cancer--From theory to practice. Biomed Pharmacother. 2021;137:111381.
[https://doi.org/10.1016/j.biopha.2021.111381]
-
Ni M, Wang H, Wang M, Zhou W, Zhang J, Wu J, et al. Investigation on the Efficiency of Chinese Herbal Injections for Treating Non-small Cell Lung Cancer With Vinorelbine and Cisplatin Based on Multidimensional Bayesian Network Meta-Analysis. Front Pharmacol. 2021;11:631170.
[https://doi.org/10.3389/fphar.2020.631170]
-
Chen RL, Wang Z, Huang P, Sun CH, Yu WY, Zhang HH, et al. Isovitexin potentiated the antitumor activity of cisplatin by inhibiting the glucose metabolism of lung cancer cells and reduced cisplatin-induced immunotoxicity in mice. Int Immunopharmacol. 2021;94:107357.
[https://doi.org/10.1016/j.intimp.2020.107357]
-
Joh EH, Hollenbaugh JA, Kim B, Kim DH. Pleckstrin homology domain of Akt kinase: a proof of principle for highly specific and effective non-enzymatic anti-cancer target. PLoS One. 2012;7(11):e50424.
[https://doi.org/10.1371/journal.pone.0050424]
-
Du YE, Lee JS, Kim HM, Ahn JH, Jung IH, Ryu JH, et al. Chemical constituents of the roots of Codonopsis lanceolata. Arch Pharm Res. 2018;41(11):1082-91.
[https://doi.org/10.1007/s12272-018-1080-9]
-
Li W, Xu Q, He YF, Liu Y, Yang SB, Wang Z, et al. Anti-Tumor Effect of Steamed Codonopsis lanceolata in H22 Tumor-Bearing Mice and Its Possible Mechanism. Nutrients. 2015;7(10):8294-307.
[https://doi.org/10.3390/nu7105395]
-
Jiang N, Dai Q, Su X, Fu J, Feng X, Peng J. Role of PI3K/AKT pathway in cancer: the framework of malignant behavior. Mol Biol Rep. 2020;47(6):4587-629.
[https://doi.org/10.1007/s11033-020-05435-1]
-
Tan AC. Targeting the PI3K/Akt/mTOR pathway in non-small cell lung cancer (NSCLC). Thorac Cancer. 2020;11(3):511-8.
[https://doi.org/10.1111/1759-7714.13328]
-
Ghomlaghi M, Hart A, Hoang N, Shin S, Nguyen LK. Feedback, Crosstalk and Competition: Ingredients for Emergent Non-Linear Behaviour in the PI3K/mTOR Signalling Network. Int J Mol Sci. 2021;22(13):6944.
[https://doi.org/10.3390/ijms22136944]
-
Conciatori F, Bazzichetto C, Falcone I, Ciuffreda L, Ferretti G, Vari S, et al. PTEN Function at the Interface between Cancer and Tumor Microenvironment: Implications for Response to Immunotherapy. Int J Mol Sci. 2020;21(15):5337.
[https://doi.org/10.3390/ijms21155337]
-
Kang BW, Chau I. Molecular target: pan-AKT in gastric cancer. ESMO Open. 2020;5(5):e000728.
[https://doi.org/10.1136/esmoopen-2020-000728]
-
Ribatti D, Tamma R, Annese T. Epithelial-Mesenchymal Transition in Cancer: A Historical Overview. Transl Oncol. 2020;13(6):100773.
[https://doi.org/10.1016/j.tranon.2020.100773]
-
Ahn JH, Jang DS, Choi JH. Lancemaside A Isolated from the Root of Codonopsis lanceolata Inhibits Ovarian Cancer Cell Invasion via the Reactive Oxygen Species (ROS)-Mediated p38 Pathway. Am J Chin Med. 2020;48(4):1021-34.
[https://doi.org/10.1142/S0192415X20500494]
-
Liu Y, Zou X, Sun G, Bao Y. Codonopsis lanceolata polysaccharide CLPS inhibits melanoma metastasis via regulating integrin signaling. Int J Biol Macromol. 2017;103:435-40.
[https://doi.org/10.1016/j.ijbiomac.2017.05.093]
- So J, Kim J. Effects of Codonopsis lanceolata extracts on bFGF-induced angiogenesis in vitro. Korean J Biotechnol Bioeng. 2003;18(1):25-9.
-
Lee SY, Ju MK, Jeon HM, Lee YJ, Kim CH, Park HG, et al. Reactive oxygen species induce epithelial‑mesenchymal transition, glycolytic switch, and mitochondrial repression through the Dlx‑2/Snail signaling pathways in MCF‑7 cells. Mol Med Rep. 2019;20(3):2339-46.
[https://doi.org/10.3892/mmr.2019.10466]
-
Lin K, Baritaki S, Militello L, Malaponte G, Bevelacqua Y, Bonavida B. The Role of B-RAF Mutations in Melanoma and the Induction of EMT via Dysregulation of the NF-κB/Snail/RKIP/PTEN Circuit. Genes Cancer. 2010;1(5):409-20.
[https://doi.org/10.1177/1947601910373795]
-
Xu W, Yang Z, Lu N. A new role for the PI3K/Akt signaling pathway in the epithelial-mesenchymal transition. Cell Adh Migr. 2015;9(4):317-24.
[https://doi.org/10.1080/19336918.2015.1016686]
-
Wu W, Wang Y, Li H, Chen H, Shen J. Buyang Huanwu Decoction protects against STZ-induced diabetic nephropathy by inhibiting TGF-β/Smad3 signaling-mediated renal fibrosis and inflammation. Chin Med. 2021;16(1):118.
[https://doi.org/10.1186/s13020-021-00531-1]
-
Zhang L, Zhou F, ten Dijke P. Signaling interplay between transforming growth factor-β receptor and PI3K/AKT pathways in cancer. Trends Biochem Sci. 2013;38(12):612-20.
[https://doi.org/10.1016/j.tibs.2013.10.001]
-
Kaufhold S, Bonavida B. Central role of Snail1 in the regulation of EMT and resistance in cancer: a target for therapeutic intervention. J Exp Clin Cancer Res. 2014;33(1):62.
[https://doi.org/10.1186/s13046-014-0062-0]
-
Sharma SV, Bell DW, Settleman J, Haber DA. Epidermal growth factor receptor mutations in lung cancer. Nat Rev Cancer. 2007;7(3):169-81.
[https://doi.org/10.1038/nrc2088]
-
Zhang X, Liu G, Kang Y, Dong Z, Qian Q, Ma X. N-cadherin expression is associated with acquisition of EMT phenotype and with enhanced invasion in erlotinib-resistant lung cancer cell lines. PLoS One. 2013;8(3):e57692.
[https://doi.org/10.1371/journal.pone.0057692]
-
Weng CH, Chen LY, Lin YC, Shih JY, Lin YC, Tseng RY, et al. Epithelial-mesenchymal transition (EMT) beyond EGFR mutations per se is a common mechanism for acquired resistance to EGFR TKI. Oncogene. 2019;38(4):455-68.
[https://doi.org/10.1038/s41388-018-0454-2]